It may be the ultimate fuel for space travel,
but right now antimatter is fleeting, difficult to work with, and
measured in atoms not pounds!
by Ron Koczor
What do you think of when you hear the
word "antimatter?" Something exotic, something unreal? Something about
your Chief Engineer not being able to keep the containment fields
up during battle?
Well, to a few scientists and university
researchers, antimatter may just be the future of human space travel.
When it comes to packing a punch, antimatter/matter reactions can't
be beat. When a particle and its antiparticle meet, they annihilate
each other and their entire mass is converted into pure energy.
Many physics textbooks describe matter
as something "that takes up space and has mass." Every physical
object that you've ever seen consists of matter. So if everything
you know is made of matter, then what's antimatter? Let's go back
to the 1930s to find an answer.
In 1928, the British physicist Paul
A.M. Dirac (1902-1984) formulated a theory for the motion of electrons
in electric and magnetic fields. Such theories had been formulated
before, but what was unique about Dirac's was that his included
the effects of Einstein's Special Theory of Relativity. Dirac's
equations worked exceptionally well, describing many attributes
of electron motion that previous equations could not.
But his theory also led to a surprising
prediction that the electron must have an "antiparticle," having
the same mass but a positive electrical charge (the opposite of
a normal electron's negative charge). In 1932 Carl Anderson observed
this new particle experimentally and it was named the "positron."
This was the first known example of antimatter. In 1955 the antiproton
was produced at the Berkeley Bevatron, and in 1995 scientists created
the first anti-hydrogen atom at the CERN research facility in Europe
by combining the anti-proton with a positron (the normal hydrogen
atom consists of one proton and one electron). But when these antihydrogen
atoms are produced, they are travelling at nearly the speed of light
and don't last too long (40 nanoseconds is typical).
Dirac's equations predicted
that all of the fundamental particles in nature must have a corresponding
"antiparticle." In each case, the masses of the particle and antiparticle
are identical, and other properties are nearly identical. But in
all cases, the mathematical signs of some property are reversed.
Antiprotons, for example, have the same mass as a proton but the
opposite electric charge. Since Dirac's time, scores of these particle-antiparticle
pairings have been observed. Even particles that have no electrical
charge, such as the neutron, have antiparticles. These have other
properties with a sign (such as magnetic moment) that can be reversed.
Lab. for Energetic Particle Science
A Penning
Trap
Penning
traps use a combination of low temperatures and electromagnetic
fields to store antimatter. While the traps can only
store incredibly small quantities, the traps will help
in developing the technologies needed for advanced propulsion
concepts.
|
|
|
Interestingly, there is no real difference
between particles and antiparticles in particle physics theories.
They are equivalent. Most theoreticians believe that at the time of
the Big Bang antiparticles and particles were created in almost equal
numbers. But why, then, is antimatter so rare today?
The tentative answer (and it is tentative,
since this question is a topic of on-going research) is in the word
almost. Present theory suggests that if particles outnumbered
antiparticles in the Big Bang by as little as one part in 100 million,
then the present universe could be explained by those extra particles
that were not annihilated by an antiparticle counterpart. Other
theories suggest that even if identical amounts of antimatter and
matter were created in the Big Bang, the physics of antimatter and
matter are slightly different. This hypothesized difference would
favour residual matter after all original antimatter had been annihilated.
So that's what antimatter is. Are
we sure that there is no antimatter left in the universe?
Dr. Charles Meegan, an astrophysicist
at the Marshall Space Flight Centre, noted that orbiting gamma-ray
observatories have measured the sky in the range of energies that
would have detected the telltale signature of antimatter annihilation.
"None of the instruments flown to date have uncovered evidence for
vast amounts of antimatter in the universe," says Meegan.
There is evidence that very energetic reactions are taking place
in isolated spots -- in the cores of some galaxies and quasars,
for example -- that create antimatter which then annihilates. But
this is not thought to be residual antimatter left over from the
Big Bang.
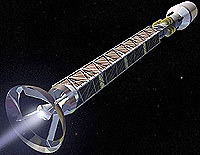
Laboratory for Energetic Particle Science
This artist's
concept of an antimatter-powered rocket ship looks like a
big space-borne linear accelerator.
|
On Earth all antimatter that exists
is counted in individual atoms. Low energy positrons are routinely
used in a medical imaging technique called Positron Emission Tomography
as well as studies of important materials used in electronics circuits.
These positrons are the result of the natural decay of radioactive
isotopes. While useful in medical and materials research applications,
there are not enough of these anti-electrons to provide a useful
form of rocket fuel. High-energy antimatter particles are only produced
in relatively large numbers at a few of the world's largest particle
accelerators. The current worldwide production rate of antimatter
is on the order of 1 to 10 nanograms (billionths of a gram!) per
year.
How can antimatter help human exploration
of space? The answer lies in Einstein's famous equation E=mc2.
When antimatter annihilates normal matter, all the mass is converted
to energy. The energy output per unit particle vastly exceeds the
efficiency of chemical reactions such as burning hydrogen and oxygen
in the Space Shuttle main engines. So, could an antimatter future
lie ahead for space travel?...
Now read our follow up Article to this
Reaching for the Stars
|