Astronomers have long thought that supernovas
are the source of cosmic rays, but there's a troubling discrepancy
between theory and measurements. Could a balloon flight over Antarctica
have shed new light on the mystery?
by Dr Tony Phillips
Hold out your hand for 10 seconds.
A dozen electrons and muons just zipped unfelt through your palm.
The ghostly particles are what scientists call "secondary cosmic
rays" - subatomic debris from collisions between molecules high
in Earth's atmosphere and high-energy cosmic rays from outer space.
Cosmic rays are atomic nuclei and electrons
that streak through the Galaxy at nearly the speed of light. The
Milky Way is permeated with them. Fortunately, our planet's magnetosphere
and atmosphere protects us from most cosmic rays. Even so, the most
powerful ones, which can carry a billion times more energy than
particles created inside atomic accelerators on Earth, produce large
showers of secondary particles in the atmosphere that can reach
our planet's surface.
Where do cosmic rays come from? Scientists
have been trying to answer that question since 1912, when Victor
Hess discovered the mysterious particles during a high altitude
balloon flight over Europe. Galactic cosmic rays shower our planet
from all directions. There's no definite source astronomers can
pinpoint, although there is a popular candidate.
"Most researchers are betting that
cosmic rays come from supernova explosions," says Jim Adams of the
NASA/Marshall Space Flight Centre. When massive stars explode they
blast their own atmospheres into space. The expanding shock waves
can break apart interstellar atoms and accelerate the debris to
cosmic ray energies. Cosmic rays are subsequently scattered by interstellar
magnetic fields -- they wander through the Galaxy, losing their
sense of direction as they go.
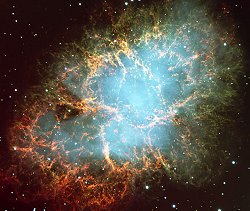
Supernova explosions,
like the one that created the expanding Crab Nebula (pictured),
may be the
source of galactic cosmic rays.
|
"It takes an awful lot of power to
maintain the galactic population of cosmic rays," says Adams. "Cosmic
rays that lose their energy or leak out of the Galaxy have to be
replenished. Supernovae can do the job, but only if one goes off
every 50 years or so." The actual supernova rate is unknown. Observers
estimate that one supernova explodes somewhere in the Galaxy every
10 to 100 years - just enough to satisfy the energy needs of cosmic
rays.
But there could be a problem with the
supernova theory, says Adams.
"A supernova blast blows a bubble in
the interstellar medium that grows until the shock wave runs out
of energy," he explained. "They can accelerate particles up to some
point, about 1014 electron volts (eV) per nucleon, but
not beyond that. Below that level all of the different cosmic ray
species - protons, helium nuclei, etc; should have the same kind
of energy spectrum: a power law with index around -2.7."
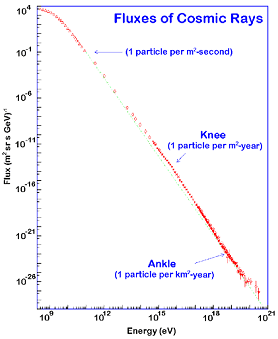
This graph
shows the flux of cosmic rays bombarding Earth as a function
of their energy per particle. Researchers believe cosmic rays
with energies less than ~3x1015 eV come from supernova
explosions. The origin of cosmic rays much more energetic
than that (above the "knee" in the diagram) remain a mystery.
|
A "power law" spectrum is one that
looks like a straight line on a piece of log-log graph paper. In
the energy range ~1010 eV to 1014 eV, the
supernova theory of cosmic ray acceleration predicts that the power
law spectrum of protons should have the same slope as the power
law spectra of heavier nuclei (about -2.7).
The problem is when scientists compare
the energy spectra of protons and helium nuclei, the two don't resemble
one another as much as they should. Both are power laws, as expected,
but "existing data indicate a possible spectral index difference
between protons and helium of about 0.1," says Eun-Suk Seo, a cosmic
ray researcher at the University of Maryland. "The [slope of the]
proton spectrum is close to -2.7, but the energy spectra of helium
and heavier nuclei seem to be flatter. The difference is small and
it might not be statistically significant." If there is a genuine
discrepancy, she added, it could signal trouble for supernova models
of cosmic ray acceleration.
To find out if the supernova theory
is indeed in peril, a team of scientists led by John Wefel ( Louisiana
State University) and Eun-Suk Seo, and aided by personnel from the
National Science Balloon Facility, launched a helium-filled balloon
from McMurdo, Antarctica on Dec. 28, 2000. The payload, which soared
to120,000 feet above Earth's surface, included a NASA-funded cosmic
ray spectrometer known by its builders as the Advanced Thin Ionisation
Calorimeter or "ATIC" for short.
"ATIC is sensitive to cosmic rays with
energies between ~1010eV and 1014eV," says
Wefel. By covering such a wide range of energies with a single modern
spectrometer, the team hopes to measure the proton and helium cosmic
ray spectra with better precision than ever before.
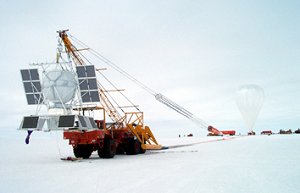
The ATIC payload
hangs from a launch vehicle while the helium balloon is being
filled in the background by personnel from the National Science
Balloon Facility.
|
The higher energy cosmic rays are rare,"
he continued. "For example, each day ATIC collects no more than ~10
cosmic rays with energies exceeding 1013 eV. That's why
we have to fly the balloon for such a long time, to gather enough
particles for a statistically significant result." By the time ATIC
landed in mid-January 2001, the spectrometer had been in the stratosphere
counting cosmic rays for nearly two full weeks.
The long flight time, more than any
other reason, is why the researchers chose to fly the balloon over
Antarctica. "We would be happy to fly this payload over North America,"
says Adams. "The problem is that we needed the spectrometer to be
aloft for a long time. Antarctica has two advantages: It's international
territory, so we don't need to apply for lots of overflight permissions,
and the Antarctic Vortex (a circulating weather system around the
south pole) keeps the balloon confined to airspace over the continent."
"If there is a difference between the
proton and the helium spectra - and that's not certain, by the way
- it won't necessarily kill the supernova model," continued Wefel.
"But a discrepancy would cause problems." Theorists may have to
consider the progress of supernova shock fronts in greater detail.
"Every supernova explosion is an individual work of art," says Adams.
"We use mathematical models that assume the explosions are spherical,
but they are not. Within the blast wave itself you can see irregularities.
There are bright knots, for example, where shock waves run into
interstellar clouds. In crowded groups of massive stars ('OB associations')
where supernovae can occur in quick succession, blast waves collide
with other blast waves." It can get a little messy! Modelling such
details might affect any necessary reconciliation between the theory
and the data.
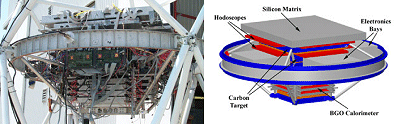
The ATIC balloon
payload.
|
And what if the supernova model can't
be rescued? "There are other possibilities," says Wefel, "but not
a lot of good ones. We'll really have to look hard to find something
other than supernovae that can meet the cosmic ray energy requirement."
The analysis team led by Eun-Suk Seo
hope that the new particle count will be the most accurate to date
in Alice's energy range, and could shed new light on the decades-old
mystery of cosmic rays.
|