If we are to get to travel to distant regions
of space and time then first we need to get to grips with that most
exotic of phenomena
the Black Hole
By Sir Martin Rees, Astronomer
Royal
Ever since the beginning, gravity has
been making our universe less and less uniform and building up ever-larger
contrasts of density and temperature. In the end, gravity overwhelms
all the other forces in stars, and in anything larger, even though
the effects of rotation and nuclear energy delay its final victory.
There are some entities in which gravity
has already triumphed over all other forces. These are black holes
- objects that have collapsed so far that no light or any other
signal can escape them, but that nonetheless leave imprints, distortions
of space and time, frozen in the space they've left.
An Astronaut who ventured too close
to a black hole would pass into a region from which there is no
return and from where no light signals can be transmitted to the
external world; it is as though space itself were being sucked inward
faster than light moves through it. An external observer would never
witness the falling astronaut's final fate: any clock would appear
to run slower and slower as it fell inward, into the hole, so the
astronaut would appear impaled at a horizon, frozen in time.
The Russian theorists Yakov Zeldovich
and Igor Novikov, who studied how time was distorted near collapsed
objects, coined the term 'frozen star' for such objects. Zeldovich,
one of the last polymaths of physics, holds a prominent place in
modern cosmology. He was a dynamic and charismatic personality;
from the 1960s onward, his research school in Moscow spearheaded
many key discoveries (even though cosmology and relativity had previously
been ideologically tainted in the USSR). The term 'black hole' itself
was not coined until 1968, when John Wheeler described how an infalling
object 'becomes dimmer millisecond by millisecond
light and
particles incident from outside
go down the black hole only
to add to its mass and increase its gravitational attraction."
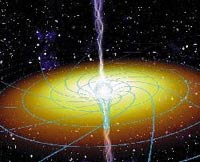
Artists impression
of a Black Hole - Possibly as heavy as 2.6 million Suns!
|
Black holes, the most remarkable consequences
of Einstein's theory, are not just theoretical constructs. There
are huge numbers of them in our Galaxy and in every other galaxy,
each being the remnant of a star and weighing several times as much
as the Sun. There are much larger ones, too, in the centres of galaxies.
Near our own galactic centre, stars are orbiting ten times faster
than their normal speeds within a galaxy. They are feeling, close
up, the gravity of a dark object, presumably a black hole, as heavy
as 2.6 million suns. Yet our Galaxy is poorly endowed compared to
some others, in whose centres lurk holes more massive than a billion
suns, betraying their presence by the high speed motions of surrounding
stars and gas, induced by their gravitational pull.
Black holes are among the most exotic
entities in the cosmos. But they are actually among the best understood.
They are constructed from the fabric of space itself and are as
simple in structure as elementary particles. A newly formed black
hole quickly settles down to a standardised stationary state characterised
stationary state characterised by just two numbers: those that measure
its mass and its spin. (In principle, electric charge is a third
such number, but stars can never acquire enough electric charge
for this factor to be relevant to real collapse). The distorted
space and time around black holes is described exactly by a solution
of Einstein's general relativity equations that was first discovered
in 1963 by Roy Kerr, a mathematician who later forsook research
to become an internationally recognised bridge player. In general,
macroscopic objects seem more and more complicated as we view them
closer up, and we can't expect to explain their every detail; but
black holes are an exception to this rule.
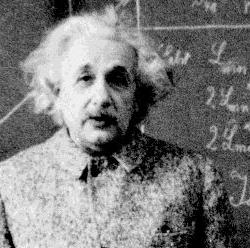
Cal Tech
Albert Einstein
|
Viewed from outside, no traces remain
that distinguish how a particular hole formed, nor what kind of
object it swallowed. The great Indian astrophysicist Subrahmanyan
Chandrasekhar was deeply impressed by this realisation, aesthetically
as well as scientifically: " In my entire scientific life,"
he wrote, "the most shattering experience has been the realisation
that an exact solution of Einstein's equations of general relativity,
discovered by the New Zealand mathematician Roy Kerr, provides the
absolutely exact representation of untold numbers of massive black
holes that populate the Universe." Roger Penrose, the theorist
who perhaps did most of to stimulate the renaissance in relativity
theory that occurred in the 1960s, has remarked. "It is ironic
that the astrophysical object which is strangest and least familiar,
the black hole, should be the one for which our theoretical picture
is most complete". The discovery of black holes thus opened
the way to testing the most remarkable consequences of Einstein's
theory.
Black Holes interest astronomers because
the flow patterns and magnetic fields around them generate some
of the most spectacular pyrotechnics in the universe. But they challenge
basic physics as well. Around any black holes is a horizon, a surface
shrouding from view an interior from which not even light can escape.
A hole's size is proportional to its mass: if the sun became a black
hole, its radius would be 3 kilometres, but some of the supermassive
holes in galactic centres are as big as our whole solar system.
If you fell inside one of these monster holes, you would be treated
to several hours of leisurely observation before you approach the
centre, where increasingly violent tidal forces would shred you
apart. Right at the centre, you, or your remains, would encounter
the singularity where the physics transcends what we yet understand.
The new physics that we'll need is the same that governs the initial
instants of the Big Bang.
Fast-Forward and Backward in Time?
Good science fiction should respect
the fundamental constraints of physical law. In that sprit, it is
worth mentioning that an observer could, in principle, observe the
far future in what, subjectively, seemed quiet a short time. According
to Einstein, the speed of a clock depends on where you are and how
you're moving. If your subjective clock ran very slowly compared
to the cosmic clock, you could travel "fast forward" into
the future. This would happen if you were moving at a velocity close
to the speed of light. Furthermore, strong gravity would distort
time; clocks on a neutron star would run 20 or 30 percent slower.
Near a black hole, the distortions would be even greater. If you
were to fall into one, your future would be finite; you would be
ripped apart - spaghettified - by ever more violent gravitational
forces. However, a more prudent astronaut who managed to get into
the closest possible orbit around a rapidly spinning hole without
falling into it would also have interesting experiences, space-time
is so distorted there that his clock would run arbitrarily slow
and he could, therefore, in a subjectively short period, view an
immensely long future timespan in the external universe.
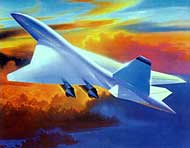
Nasa
Non Stop luxury
- HSCT - The successor to Concorde - While not fast enough
for time travel; it will be capable of flying over 200 passengers
at Supersonic speeds over the Atlantic.
|
This elasticity in the rate of passage
of time may seem counter to our intuition. But such intuition is
acquired from our everyday environment (and perhaps, even more,
that of our remote ancestors), which has offered us no experience
of such effects. Few of us have travelled faster than a millionth
of the speed of light (the speed of a jet airliner); we live on
a planet where the pull of gravity is 1000 billion times weaker
than on a neutron star. But time dilation entails no inconsistency
or paradox.
More problematic, of course, would
be time travel back into the past. More than fifty years ago, the
great logician Kurt Godel discovered that the theory of general
relativity did not in itself preclude a time machine. He discovered
a valid solution of Einstein's equations that described a bizarre
universe where some of the worldlines were close loops - in other
words, you could come back into your own past. But Godel's solution
was not realistic: it described a universe that was rotating and
not expanding.
Other theoretical examples of systems
that seem to obey the laws of physics but which allow closed loops
in time have been proposed. For example, Princeton theorist Richard
Gott showed that a time machine could be constructed from two so
called cosmic strings - long microscopically thin tubes of hyperdense
material, heavy enough to distort space. Gott and his colleague
Li-Xin Li also devised a cosmological model even stranger than Godel's
in which an entire universe, with a finite life cycle, traces out
a loop in time so that its end is also its beginning.
One much-discussed design for a time
machine involves a "wormhole": two black holes linked
together by a tunnel or "spacewarp". The tunnel could
exist only if it were made of a substance that has very large negative
pressure (or tension). Theorists speculate that exotic stuff of
this kind did exist in the early universe, but even if such material
still existed, the mass needed in order to make a wormhole wide
enough to be comfortably traversed by a human would be 10,000 times
that of the Sun!
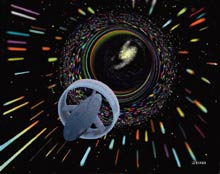
Wormhole Travel?
|
Godel's discovery and its aftermath
opened up a debate. Is there a future law of physics, more restrictive
than Einstein's equations that rule out such effects? One might
call it a "chronology protection law". Or could a time
machine in principle exist? Such an artefact plainly still lies
in the hypothetical reaches of science fiction, but we can still
ask whether the barriers to constructing a time machine are merely
technological, or whether there is a fundamental physical law that
prohibit them. (To clarify the distinction, most physicists would
say that a large spaceship travelling at 99.99 percent of the speed
of light is in the first category, but one that travels faster than
light is in the second.)
The events on the time loop must close
up self-consistently, as in a movie whose last scene recapitulates
its first. Paradoxes arise if you come back into the past and undo
something that was a precondition of your existence: for instance,
murdering your grandmother in her cradle would raise issues of logical
consistency, not just of ethics. Time travel makes sense only if
some law of nature precludes inconsistency of this kind. The implication
that there must be "time police" to constrain our free
will might seem paradoxical. But I am convinced by the robust retort
of Igor Novikov, a leading physicist who has explored these ideas,
that physical laws already constrain our choices: we cannot, for
instance, exercise our free will by walking on the ceiling. The
prohibition on violating the consistency of a time loop is, in a
sense, analogous.
Even if a time machine could be built,
it would not enable us to travel back prior to the date of its construction.
So the fact that we have not been invaded by tourists from the future
may tell us only that no time machine has yet been made, not that
it is impossible.
An Abridged extract from 'Our
Cosmic Habitat' by Martin Rees(C) Published
by Princeton University Press and copyrighted, © 2002,
by Princeton University Press. All rights reserved. No part
of this book may be reproduced in any form by any electronic
or mechanical means (including photocopying, recording, or
information storage and retrieval) without permission in writing
from the publisher, except for reading and browsing via the
World Wide Web. Users are not permitted to mount this file
on any network servers. By permission of Princeton
University Press ($22.55/£15.95) Published
in the UK by Weidenfeld and Nicolson (£14.99)
Available to buy from Amazon.co.uk
and Amazon.com
Martin Rees is Royal Society
Research Professor at Cambridge University, and holds the
title Astronomer Royal of Great Britain. We have previously
featured two articles by Martin -
Are
We Alone? and
Recipe
for the Universe.
|
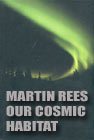 |
|
|
|