Watching liquids boil in low gravity is an out-of-this-world
experience. It has plenty of entertainment value, but it's teaching
scientists some important physics lessons, too.
by Patrick Barry
The next time you're watching a pot
of water boil, perhaps for coffee or a cup of soup, pause for a
moment and consider: what would this look like in space? Would the
turbulent bubbles rise or fall? And how big would they be? Would
the liquid stay in the pan at all?
Until a few years ago, nobody knew.
Indeed, physicists have trouble understanding the complex behaviour
of boiling fluids here on Earth. Perhaps boiling in space would
prove even more baffling.... It's an important question because
boiling happens not only in coffee pots, but also in power plants
and spacecraft cooling systems. Engineers need to know how boiling
works.
In the early 1990's a team of scientists
and engineers from the University of Michigan and NASA decided to
find out. Using a freon coolant as their liquid, they conducted
a series of boiling experiments on the space shuttle during 5 missions
between 1992 to 1996. And indeed, they found some intriguing differences
between what happens to boiling fluids on Earth and what happens
to them in orbit. For example, a liquid boiling in weightlessness
produces - not thousands of effervescing bubbles - but one giant
undulating bubble that swallows up smaller ones!
"Think of it: no one had really seen
boiling in space before these experiments - in the whole world,
ever!" says Dr. Francis Chiaramonte, who was the NASA Project Scientist
for the Pool Boiling Experiment. Already, he says, the series of
experiments has come to be regarded as "classic" by today's researchers.
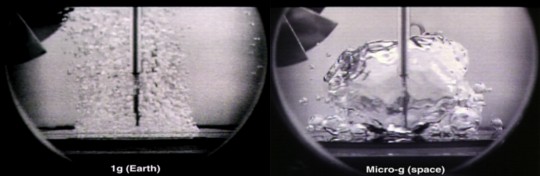
Image courtesy NASA Glenn Research Centre .
Without buoyancy
or convection, boiling fluids behave quite differently in
space.
|
Despite its entertainment value, this research is much more than
a simple curiosity. Learning how liquids boil in space will lead
to more efficient cooling systems for spacecraft, such as the ammonia-based
system on the International Space Station. Knowledge of boiling
in space might also be used someday to design power plants for space
stations that use sunlight to boil a liquid to create vapour, which
would then turn a turbine to produce electricity.
The research could also have applications
here on Earth. The weightless environment gives scientists a new
"window" into the phenomenon of boiling. Scientists can use this
perspective to improve their understanding of the fundamentals of
boiling, which might be used to improve the design of terrestrial
power plants.
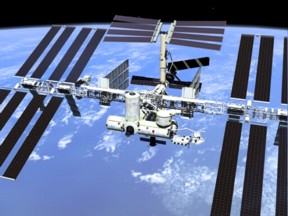
The International
Space Station uses a "2-phase" cooling system in which ammonia
changes from liquid to vapour and back, which involves boiling.
Engineers designing the ISS cooling system used information
gleaned from microgravity boiling experiments.
|
"The phenomenon of boiling is so complex
that most of our understanding is empirical, rather than based on
the solutions to fundamental equations," Chiaramonte says.
In the free-fall of orbit, boiling is simpler than it is on Earth.
Weightlessness effectively removes two of the variables in boiling
- convection and buoyancy. This difference explains why boiling
liquids behave so differently in space. It also provides a powerful
tool for scientists who want to unravel the tangled physics of boiling.
"As an example, imagine you were trying to study the Earth, which
has such complex ecosystems. You would also want to look at a simpler
planet with fewer variables. One thing space does for us is simplify
the problem that we're studying," Chiaramonte says.
When a pool of liquid is heated on
Earth, gravity causes hotter regions in the liquid to rise, and
cooler, more dense parts to sink - a process called "convection."
This motion spreads the heat around inside the liquid. Once it begins
to boil, buoyancy sends bubbles hurling upward, creating a "rolling
boil."
All of this motion within the liquid makes the physics of the situation
much more complex.
Without convection or buoyancy, the
process unfolds differently. Heated fluid doesn't rise, and instead
just sits next to the heater surface and continues to get warmer.
Regions of liquid away from the heater remain relatively cool. Because
a smaller volume of water is being heated, it comes to the boil
much more quickly. As bubbles of vapour form, though, they don't
shoot to the surface - they coalesce into a giant bubble that wobbles
around within the liquid.
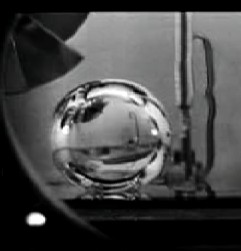
Without buoyancy,
the vapour produced by boiling simply floats as a bubble inside
the liquid after the heating has stopped. Surface tension
effects cause the many small bubbles produced to coalesce
into one large sphere.
|
Much of this could be predicted from existing theory, but to learn
the fine details of the process and to look for unexpected behaviours,
a real experiment was necessary.
"There were many fundamental issues
that were still not understood well," says Dr. Herman Merte, the
Principal Investigator for the experiments. Merte, who some see
as a kind of "founding father" of microgravity pool boiling research,
devised the experiments.
Merte and other scientists had performed
earlier research on weightless boiling using "drop towers," which
could simulate zero-G for a few seconds by simply dropping samples
inside a tall tower. These early experiments provided some guidance
for designing the shuttle-based experiment, but these brief glimpses
don't really compare to the minutes-long observation provided by
the shuttle.
One important product of that early
research, though, was a method for building a boiling chamber that
let scientists look through the heater surface and watch the liquid
right where it contacts the heater.
"The action is right at the solid-liquid interface at the heater,
and you can't look down from the top because you have the refraction
of the fluid's upper surface that interferes," says Merte, who recently
retired as Emeritus Professor of Mechanical Engineering at the University
of Michigan.
Image courtesy NASA Glenn Research Centre
One way to
simulate the weightlessness of space is to simply let an experiment
freefall inside a "drop tower," such as this one at NASA's
Glenn Research Centre in Cleveland, Ohio. Other
methods for simulating weightlessness include flying parabolic
arcs in aircraft - such as NASA's KC-135 "vomit comet" used
to train astronauts - and using sounding rockets.
|
Merte used quartz to make a smooth,
hard, transparent bottom for the boiling chamber. Then he coated
that quartz with an ultra-thin layer of gold. Less than 400 angstroms
thick (an angstrom is one ten-billionth of a meter), this layer
was so thin that it allowed visible light to pass through it, yet
it still conducted electricity like bulk gold.
Using this apparatus, Merte and his
colleagues made some interesting discoveries. For example, depending
on the temperature conditions of the experiment, the giant bubble
would sometimes float in the centre of the liquid and sometime remained
attached to the heater surface. When the bubble remained attached
- which Merte calls a "dry out" - it effectively insulated the
liquid from the heater, preventing further boiling and causing the
heater temperature to soar.
Knowing precisely the conditions when this occurs is vital for designing
spacecraft systems that might rely on boiling.
"If you understand a phenomenon better, then you can design for
it closer to its limits for optimisation," Merte says. "If you have
an uncertainty, then you're going to design conservatively."
Today's researchers continue to expand
on the foundation of knowledge laid by these experiments. With a
better understanding of the physics of boiling fluids, engineers
will be able to design improved cooling and power systems to serve
people in the future - both in space and here on Earth.
|