Here's something to ponder over your next
cup of coffee. The physics of a humble bag of ground coffee still
holds surprises for scientists...
by Patrick L. Barry and Dr.
Tony Phillips
Perhaps you wonder about it - just for a moment - each
time you open a fresh brick of vacuum-packed coffee to make that
coveted morning mug.
Remarkable, you might think to yourself,
how hard and strong the brick is before the "ssss" sound
of cutting it open, and how quickly it becomes soft and pliable
afterward. It's as if the coffee grounds themselves have transformed:
A solid one moment, a powder the next.
Why does this happen?
Coffee grains have jagged irregular shapes.
(Look at some through a magnifying glass and you'll see.) In a vacuum-packed
bag, the pressure exerted inward by the atmosphere squeezes the
coffee grounds from all sides; their odd shapes interlock to help
hold them in place. Because each particle fiercely resists motion,
the brick of coffee as a whole will be rigid. When the bag is opened
and the pressure relaxes, the coffee grounds can tumble and flow
like a powder.
Simple. Yet physicists cannot predict from theory
exactly how hard a vacuum-packed bag of coffee should be … or when
it will change from a solid to a powder.
There's no mystery to an individual coffee ground.
We can readily determine its chemical composition, its jagged shape,
its density, its crystal structure, and so on. Individual grains
are not the problem. It's the millions of individual grains rubbing
together that are so hard to predict.
Coffee is an example of a granular
material - substances that are as common as the sand beneath your
feet, but which have no complete physical theory to describe their
behaviour. NASA is interested in granular materials for several
reasons.
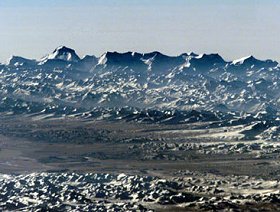
Credit Nasa
The towering
Dhaulagiri Range of the Himalayas in central Nepal as shown
here in a photo from the International Space Station. Like
all mountain ranges; at times they will experience avalanches,
which show a more devestating example of the granular effect.
|
"It's likely that large amounts of
granular materials will have to be processed in order to provide
oxygen and fuel for humans on Mars and the Moon," explains physics
professor James Jenkins, a researcher at Cornell University. "Also
granular flows are important in the formation of geological features
such as dunes and avalanche deposits seen on distant planets and
moons. A better understanding of grain flow could provide an indication
of the conditions under which those features were formed." Planetary
rings are granular, too, and astronomers would like to understand
them better as well.
"Granular flows are ubiquitous on Earth,"
adds Jenkins. "Avalanches of rock and granular snow are two examples.
Flows of granular materials that resemble avalanches are important
in coal-fired power plants, in the manufacture of pharmaceuticals,
in the processing of aluminium, and in the production of plastics
from pellets. It's hard to think of an industry that does not employ
a granular flow during some processing operation."
Unfortunately, the physics of granular
materials doesn't boil down to simple equations as easily as some
other phenomena. The helium in a balloon, for example, is also made
of many millions - in fact, billions of trillions - of particles.
Yet one simple equation governs all of its important traits: pressure,
volume and temperature. (Remember "PV=nRT"
from high school physics?)
The difference is that the helium atoms are
widely separated (on a molecular scale). One helium atom is mostly
identical to any other. There are no irregular edges or complicated
atom-to-atom interactions. It really is simple.

Image courtesy NASA.
Electron
micrographs of irregularly-shaped sand grains.
|
In a bag of coffee, however, the grounds
bump, rub, and press against each other. Each grain is unique and
it interacts strongly with its neighbours. Because these interactions
can't reasonably be ignored, the coffee must be considered as more
than just the sum of its parts. Instead, it is the sum of its parts
plus their interactions!
Computers are ideal for solving such problems,
but there's a snag: There are enough interactions in a single bag
of coffee to overwhelm a supercomputer.
When scientists and engineers need to deal with
granular materials like soils and powders, they usually approach
the problem empirically - that is, they measure how the material
behaves in real life and make predictions accordingly. But the empirical
approach is limited to things easily measured. Some things aren't.
For example, what triggers avalanches on the Moon? How much
soil can flow down a chute on Mars? Or, right here on Earth,
what happens to damp sand underneath a building during an earthquake?
To answer such questions we need a theory, a "PV=nRT" for granular
flows, that can make predictions under a wide range of circumstances.
NASA-supported researchers are working to develop
such a theory through a combination of experimentation and mathematics.
Jenkins, for example, is studying differential equations that describe
molecular gases. It might be possible, he says, to adapt them for
granular flows. He plans to test some of his ideas using a rotating
chamber filled with beads; the device is slated for launch to the
International Space Station (ISS) in 2007.

A sand
column is compressed during an MGM experiment onboard shuttle
flight STS-79. The speed of the movie is misleading; the
complete sequence takes about an hour.
|
"We do this on the ISS," he explains, "because
granular flows are affected by both gravity and internal collisions.
We need to get Earth's gravity out of the picture to create a simpler
system."
For the same reason, engineering professor
Stein Sture of the University of Colorado is leading a series of
experiments called "Mechanics of Granular Materials" (MGM) onboard
the space shuttle. His device repeatedly squeezes a column of damp
sand and records what happens. The goal, explains Sture, is to understand
the liquid-like behaviour. of soil during some earthquakes. MGM
has flown before on shuttle missions STS-79 and STS-89, and it's
slated for another flight in 2003 onboard the shuttle Columbia (STS-107).
Earthquakes, avalanches, planetary
rings, coal mines ... even bags of coffee. From the alien to the
ordinary, we'll understand them all a little better when this research
is done.
|