The physics underlying common everyday foams
is poorly understood. An experiment scheduled to fly on the International
Space Station will help fill in the gaps.
by Patrick L Barry and Dr Tony
Phillips
Put some dish-washing soap in the kitchen
sink and fill it with water. You'll create a truly bizarre substance.
Despite being made almost completely of air, the sudsy foam in that
sink somehow behaves like a springy solid. Strange.
Douglas Durian, a professor of physics
at UCLA suggests the following: "Take some shaving cream and put
it in your hand. Touch it. Run your fingers through it. Ask yourself,
is it a solid, a liquid, or a gas?"
Ordinary aqueous foams, like shaving
cream or the suds in a dishwasher's sink, are mostly gas (95%) and
a little bit of liquid (5%). The gas subdivides the liquid into
a matrix of tiny bubbles. Good foams usually contain complex molecules
that toughen the walls of the bubbles. Milk fat, for instance, serves
this purpose in whipped cream. The way the bubbles stick together
or slip past one another determines how the foam behaves.
Many of us are so accustomed to foams
that we hardly notice how odd they are. Foams are on our legs or
faces when we shave, on our dishes as we wash them, atop our glasses
of beer. "Yet the physics of foam is poorly understood," says Durian.
Much of what is known comes from trial
and error. No theory currently exists for predicting exactly how
stiff or oozy a foam will be based on its traits like the size of
its bubbles or the amount of liquid it contains. And the precise
stiffness of a foam is crucial for many uses. Just imagine: a fire-retardant
foam that must flow quickly through the valve of the extinguisher
and then cling tightly where it lands; or a counter-biological weapons
agent that expands to fill cracks and crevasses and kills microbes
hiding there.
Durian would like to take the guesswork out of foams by learning
more about their fundamental physics. That's the goal of an experiment
he and colleagues are designing for the International Space Station
(ISS). It's called FOAM, short for Foam Optics and Mechanics.
"One way to understand the basic physics
of any material is to explore its 'critical point' - the threshold
where the material changes phases, for instance, from a solid to
a liquid," says Durian. "Exploring the critical point of foams is
what FOAM will do."

Courtesy Dan Sandler
A foam near the "critical point" would have perfectly round
air bubbles
|
Foams, which can act like solids, are
part gas and part liquid. What does it mean for such a substance
to change phases?
Durian explains: The critical point
of a foam occurs when the liquid content is so high (roughly 37%
by volume) that the air bubbles are completely spherical and only
touch each other at one point, like steel ball bearings piled together
in a jar. That's when the foam ceases to act like a semi-solid stack
of bubbles and begins acting instead like bubbles floating freely
inside a flowing liquid - a "phase change" of sorts.
"It's impossible to explore the critical
point of a foam on the ground, but in space we can study it quite
well," Durian says.
Gravity causes the liquid in a foam
to ooze downward, especially when the foam is relatively wet as
it would be near the critical point. Here on Earth the critical
point can't be reached because the liquid quickly pools at the bottom
of the container, leaving a foam with odd flat-sided bubbles and
only about 5% liquid content floating on top.
"In orbit, drainage of the foam is
virtually absent, so we can bring a foam to the critical point and
then explore it at our leisure," Durian says.
How do you explore a foam? You can't
touch it, obviously, or you'll pop the bubbles and change the foam.
Somehow, the researchers need a way to measure the traits of a foam
without disturbing it.
The answer, says Durian, is light.
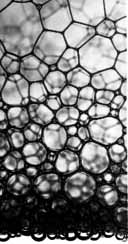
Image courtesy NASA.
The gravity-driven
flow of liquid toward the bottom of a foam prevents scientists
from experimenting near the "critical point" here on the
ground. Notice how the walls of the bubbles near the
bottom of the image - where the liquid content is greater
- are more rounded, while the bubbles higher up have straighter,
more angular sides.
|
Over roughly the last 10 years, Durian's
research group at UCLA along with others have been developing ways
to use beams of light to measure the size, wetness, and movement
of bubbles in a foam. These techniques are central to the FOAM experiment.
In one method, called "diffuse-transmission
spectroscopy," the scientists shine the beam through the foam and
measure how much of the light reaches the point on the other side.
In a foam with only a few, very large bubbles, most of the light
will pass straight through with little interference; in a foam of
many, tiny bubbles, the light will get scattered by the bubble membranes.
Measuring how much light reaches the far side lets the scientists
quantify the average bubble size.
The motion of the bubbles can also be detected using monochromatic
(single-coloured) light. As a laser beam passes through the foam,
bubble membranes in motion cause a slight Doppler effect, shifting
the frequency - and hence the colour of the light. Watching these
ever so slight shifts in the light's frequency tells researchers
how fast the bubbles are moving and in what direction. This technique
is called "diffusing-wave spectroscopy."
Onboard the ISS, a simple water-based
foam will be formed within the FOAM apparatus. Durian and colleagues,
who will be able to remotely control the experiment from the ground,
will select the ratio of liquid-to-gas so the foam is near its critical
point. Then they'll shine a laser beam through the foam to explore
its properties as the foam is twisted and deformed by mechanical
plates.
"The goal," says Durian, "is to discover
how the internal structure of the foam changes as its elastic character
vanishes." The data will be fundamental. They're bound to interest
anyone who wants to spray a foam around a corner or into a fire
... or anyone who wants to craft a physical theory of foam.
And best of all, perhaps, it's something
to think about the next time you're doing the dishes.
|