An experiment onboard the International
Space Station is helping physicists decipher the group behaviour
of atoms and molecules.
by Dr Tony Phillips
"It would indeed
be remarkable if Nature fortified herself against further advances
in knowledge behind the analytical difficulties of the many-body
problem." (Max Born, 1960)
One water molecule
all by itself isn't very interesting. One oxygen. Two hydrogen's.
What more can you say? But throw a lot of water molecules together….
You get snowflakes, rainbows, rock-hard ice, a soft cooling mist,
infinitely flexible water.
Atoms and molecules,
en masse, can do almost anything, and physicists know it. In fact,
they dream about it. By combining the right kinds of molecules under
the right conditions, it should be possible to craft, e.g.,
uncrackable metal alloys, room-temperature superconductors, self-healing
spaceship skins impervious to meteoroids and solar flares. You name
it.
The promise of "designer
materials" is great, but there's a problem: Crowds of molecules,
like crowds of people, can be hard to predict. Only in idealized
cases do physicists have simple rules, like the ideal gas law, to
help them describe many-particle systems. Sometimes those rules
work well. The wonder of designer materials, however, lies beyond
the ideal.
Physics can deal handily
with one or two particles. Newton's laws, for instance, describe
the motion of a single planet around the sun so simply and beautifully
that school children can solve the equations. Now add another planet;
all three bodies (sun, planet and planet) tug on each other as they
move. The "three body problem" is famously complicated; computers
are required to solve it exactly.
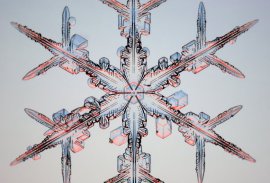
more
A Wisconsin snowflake, captured and photographed by K. Libbrecht
and P. Rasmussen.
|
There's a three-body
problem in quantum mechanics, too. Schrodinger's equation is easy
to solve for two particles, e.g., an electron and a proton
in a hydrogen atom. Add one more electron and, once again, a computer
is required.
Now imagine, not three,
but 1023 particles. That's the number of atoms or molecules
in, say, a tablespoon of water. They tug on each other, bond with
each other, crash into each other. The number of interactions going
on at any instant is mind-boggling.
"It's impossible to
solve the equations exactly for such a system," says physicist
Peter J. Lu of Harvard University. "With 1023 particles,
you have to write down a term for each interaction. So, of course,
you make approximations because the exact calculation is far, far
beyond anyone's (or any computer's) abilities."
What do you do when
even supercomputers can't handle the maths? Enter the International
Space Station (ISS).
Lu and his PhD thesis
adviser Prof. David Weitz are testing a device onboard the ISS that
might succeed, in some respects, where supercomputers fail.
It's simple: Take a
jar of "organic goop," says Lu, and mix in millions of tiny Plexiglas
spheres. Add some molecular coils, billions of them, and float the
mixture in space. This "device" is a colloidal mixture, and it's
a good model for many-particle interactions.
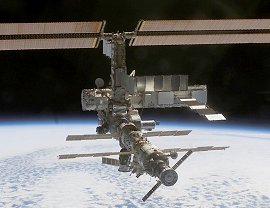
The International
Space Station
|
Colloids are systems
of tiny particles suspended in fluid. (Pulpy orange juice is an
everyday example.) Physicists have known for a long time that carefully
crafted colloids could be used to simulate swarms of atoms or molecules.
Colloidal particles arrange themselves as crystals; flow like fluids;
expand and contract like a gas. They exhibit all the mob behaviours
of molecules, with a big advantage:
"We can see colloids,"
says Lu. While it's impossible to watch individual atoms or molecules,
colloidal particles are big enough to see through an ordinary microscope.
Their interactions, and the structures they form, can be observed
directly. No supercomputer required!
On Earth colloidal
simulations are limited. The particles, weighted by gravity, tend
to settle to the bottom of the jar. Floating onboard the ISS, however,
they remain suspended, interacting for as long as a physicist cares
to watch.
What kinds of atoms
and molecules do colloids mimic?
It depends on the make-up
of the colloid. Some colloid-spheres can carry a charge, "so we
can make them attract or repel" like ions, says Lu. "We can also
mix colloids of different sizes, and vary their ratios to grow a
host of different crystal structures that mimic real materials."
The possibilities are endless.
But the research is
just beginning.
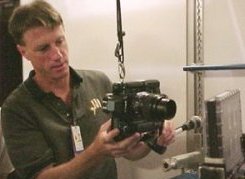
Astronaut
Mike Foale photographs colloids onboard the ISS.
|
In recent months, Weitz,
Lu and colleagues at the University of Edinburgh (led by Prof. Peter
Pusey) have been working with six simple colloids onboard the ISS.
Astronauts do the actual work; Weitz et al. send directions
from the ground. The name of the experiment is BCAT-3, short for
Binary Colloid Alloy Test-3.
One of the colloids,
Lu's favourite, is made of 400-nanometer Plexiglas spheres, polymer
coils (long molecules coiled up like a Slinky), and an organic fluid
similar to gasoline. The spheres are stand-ins for atoms or molecules.
The polymer coils force the spheres to interact. Lu explains: "Polymer
coils act like an ideal gas. They swarm through the liquid, applying
pressure to the bigger spheres. When two spheres approach one another,
the gap between them becomes too small for the coils. Inside the
gap, pressure drops and the spheres are pulled together. By controlling
the size and concentration of the polymer coils, we control the
strength of interactions between the Plexiglas spheres."
This colloid mixture
turns out to be a promising model for supercritical fluids.
A supercritical fluid
is a high-pressure, high-temperature state of matter best described
as a liquid-like gas, and a marvelous solvent. Water becomes supercritical
in some steam turbines - and it tends to dissolve the tips of the
turbine blades. Supercritical carbon dioxide is used to remove caffeine
from coffee beans, and sometimes to dry-clean clothes. Liquid-fueled
rocket propellant is also supercritical when it emerges from the
tail of a spaceship.
Of special interest
is the "critical point" - the pressure and temperature where a substance
becomes supercritical. Near the critical point, matter fluctuates.
Bubbles and droplets, some as small as a few atoms, some as wide
as the jar itself, appear and disappear, merge and split. Weitz
and Lu have been able to observe these kinds of structures in BCAT-3,
and they're developing new insights into critical phenomena.
It's only a start,
says Lu. If you want to design a supercritical fluid … good luck!
Maybe one day, though, all you'll need is a good colloid.
|