NASA researchers are developing an exquisitely sensitive
artificial nose for space exploration.
by Karen Miller
Onboard the space station,
astronauts are surrounded by ammonia. It flows through pipes, carrying
heat generated inside the station (by people and electronics) outside
to space. Ammonia helps keep the station habitable.
But it's also a poison.
And if it leaks, the astronauts will need to know quickly. Ammonia
becomes dangerous at a concentration of a few parts per million
(ppm). Humans, though, can't sense it until it reaches about 50
ppm.
Ammonia is just one
of about forty or fifty compounds necessary on the shuttle and space
station, which cannot be allowed to accumulate in a closed environment.
And then there's fire.
Before an electrical fire breaks out, increasing heat releases a
variety of signature molecules. Humans can't sense them either until
concentrations become high.
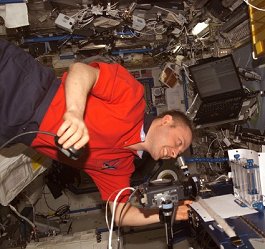
Astronaut Mike Fincke onboard the International Space Station.
|
Astronauts need better
noses!
That's why NASA is
developing the Electronic Nose, or ENose for short. It's a device
that can learn to recognize almost any compound or combination of
compounds. It can even be trained to distinguish between Pepsi and
Coke. Like a human nose, the ENose is amazingly versatile, yet it's
much more sensitive.
"ENose can detect an
electronic change of 1 part per million," says Dr. Amy Ryan who
heads the project at JPL. She and her colleagues are teaching the
ENose to recognize those compounds - like ammonia - that cannot
be allowed to accumulate in a space habitat.
Here's how it works:
ENose uses a collection of 16 different polymer films. These films
are specially designed to conduct electricity. When a substance
- such as the stray molecules from a glass of soda - is absorbed
into these films, the films expand slightly, and that changes how
much electricity they conduct.
Because each film is
made of a different polymer, each one reacts to each substance,
or analyte, in a slightly different way. And, while the changes
in conductivity in a single polymer film wouldn't be enough to identify
an analyte, the varied changes in 16 films produce a distinctive,
identifiable pattern.
Electronic Noses are
already being used on Earth. In the food industry, for example,
they can be used to detect spoilage. There's even an Electronic
Tongue, which identifies compounds in liquids. The ENose needs to
be able to detect lower concentrations than these devices.
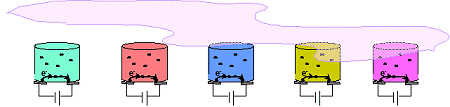
E-Nose
|
Right now, Ryan is
working on a stand-alone version of ENose. "Everything is in one
package," she explains: polymer films, a pump to pull air (and everything
in the air) through the device, computers to analyze data, the energy
source. The noses could simply be posted, like smoke detectors,
at various points around the habitat.
Ultimately, Ryan believes,
the ENose could serve as the sensory part of an intelligent safety
system. "We'd have a lot of them, connected to a central computer."
Any change in the atmosphere would set off a cascade of activity.
If the signal suggests
a fire, says Ryan, "then the crew would immediately be notified."
But if not, then the computer would try to determine exactly what
was going on. Had it detected something toxic? Had it detected something
that was approaching dangerous levels? Where is it coming from?
Depending on its answers,
the system could choose from a range of responses - from notifying
the crew, to turning on fans to change the direction of air flow,
to turning on filters, to sealing off an area.
As a safety device,
the ENose has a lot to offer here on Earth, too. With some modifications,
says Ryan, an ENose could be used to check for gas buildups in offshore
oil rigs. "The workers have to go down into the legs of the rigs,
and they want to make sure it's not going to blow up while they're
in there." Sanitation workers would benefit by knowing if any poisonous
gases have collected down in the sewers. There are many other examples.
Ryan's team is working
on an advanced version of the ENose that could expand its usefulness
even more.

The
Second Generation ENose. The volume of this design is
~760 cm3, about 35% of the original ENose.
The computer (right) can be attached to the back of the
sensor package (left).
|
"When we first started
choosing polymers for the ENose," recalls Ryan, "we used what you
might call an 'Edisonian' approach." (That's a scientist's way of
saying trial and error. Edison tried thousands of filaments before
he perfected the first light bulb.) "We tested between eighty and
one hundred polymers against each analyte." That's a lot of testing.
But, points out Ryan,
Edison's approach means that you can only use the ENose to identify
substances whose patterns are already known. Ryan and her team are
starting to go beyond that. They're trying to develop a computer
model that can predict the responses of any polymer to any analyte - "without
having to test a hundred polymers," she says. This would greatly
accelerate the pace of ENose design. Ryan's team has already made
enough progress to select some polymers using the model.
This is exciting, she
says, because a successful computer model could also be used to
help ENose identify unknown compounds.
"We want to be able
to look at an unknown response, and then figure out what caused
it," says Ryan. Such an ENose could identify unexpected vapors on
Earth or in space habitats. It could even analyze strange gases
encountered on interplanetary explorations.
Picture this: An astronaut
lands on an alien world. Strange landforms beckon in all directions.
Where to begin? Simple. "Hey, that crater smells interesting!" Follow
your ENose.
|