NASA
is building an advanced humanoid system called Robonaut, currently
under development at the Johnson Space Centre.
by Chris
Culbert
The Shape
of Things to Come
Over the past
five decades, space flight hardware has been designed for human
servicing. Space walks are planned for most of the assembly missions
for the International Space Station, and they are a key contingency
for resolving on-orbit failures. Combined with substantial investment
in EVA tools, this accumulation of equipment requiring a humanoid
shape and an assumed level of human performance presents a unique
opportunity for a humanoid system.
While the depth
and breadth of human performance is beyond the current state of
the art in robotics, NASA targeted the reduced dexterity and performance
of a suited astronaut as Robonaut's design goals, specifically using
the work envelope, ranges of motion, strength and endurance capabilities
of space walking humans. This article describes the design effort
for the Robonaut system.
Mechanism
Design
The manipulator
and dexterous hand have been developed with a substantial investment
in mechatronics design. The arm structure has embedded avionics
elements within each link, reducing cabling and noise contamination.
Unlike some systems, Robonaut uses a chordate approach to data management,
bringing all feedback to a central nervous system, where even low-level
servo control is performed. This biologically inspired neurological
approach is extended to left-right computational symmetry, sensor
and power duality and kinematical redundancy, enabling learning
and optimization in mechanical, electrical and software forms. The
theory that manufacturing tools caused humans to evolve by requiring
skills that could be naturally selected is applied to Robonaut's
design as well. The set of EVA tools used by astronauts was the
initial design consideration for the system, hence the development
of Robonaut's dexterous five-fingered hand and human-scale arm that
exceeds the range of motion of even unsuited astronauts. Packaging
requirements for the entire system were derived from the geometry
of EVA access corridors, such as pathways on the Space Station and
airlocks built for humans.
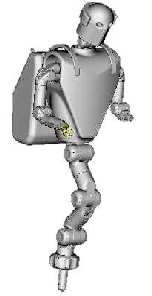
NASA
Is
this the future of robotics...?
|
Sensors and
Telepresence Control
Robonaut's broad mix of sensors includes thermal, position, tactile,
force and torque instrumentation, with over 150 sensors per arm.
The control system for Robonaut includes an onboard, real time CPU
with miniature data acquisition and power management in a small,
environmentally hardened body. Off-board guidance is delivered with
human supervision using a telepresence control station with human
tracking.
Meeting the needs
To meet the dexterous manipulation needs foreseen in future NASA
missions, the Automation, Robotics, and Simulation Division at Johnson
Space Center is developing Robonaut, a highly dexterous anthropomorphic
robotic system. Robonaut is advancing the state of the art in anthropomorphic
robotic systems, multiple use tool handling end effectors, modular
robotic systems components and telepresence control systems. The
project has adopted the design concept of an anthropomorphic robot
the size of an astronaut in a space suit and configured with two
arms, two five-fingered hands, a head and a torso. Its dexterous
pair of arms enables dual-arm operations and its hands can interface
directly with a wide range of interfaces without special tooling.
Its anthropomorphic design enables intuitive telepresence control
by a human operator.
Head
Robonaut's head is still a work in progress, but the existing system
includes an articulated neck that allows the teleoperator to point
Robonaut's face. The head holds two small color cameras that deliver
stereo vision to the operator's helmet display, yielding a form of
depth perception. The interocular spacing of the cameras is matched
to typical human eye spacing, with a fix vergence at arm's reach.
The neck drives are commanded using a 6 axis Polhemus sensor mounted
on the
NASA
The
operator can see through two cameras in Robonauts' head
|
teleoperator's
helmet, and can track the velocities of typical human neck motions.
Like the arms, the neck's endoskeleton is covered in a
fabric skin, which is fitted into and under the helmet. The helmeted
approach is unusual in the robotics world, where cameras are typically
mounted in exposed locations on pan-tilt-verge units. Robonaut's
requirements for a rugged design, working with astronauts in cluttered
environments drove the design towards a better protection system,
such as
the helmets that humans where here on Earth. The helmet is
made of an epoxy
resin, "grown" using a stereo lithography machine at the Johnson space
center. As you can see, the design was inspired by Centurian armor,
giving Robonaut some attitude. The neck joints are similar
to the joints and
are controlled with the same real-time control system. Their kinematics
is based on a pan-tilt serial chain, with the first rotation about
Robonaut's spine, and then a pitch motion about a lateral axis. The
pitch motion axis does not pass through the camera sensors, but is
instead 3 inches below, like the Atlas joint in the human neck. This
offset (actually a D-H link length) allows the cameras to translate
forward, letting Robonaut see down over its chest.
Hand
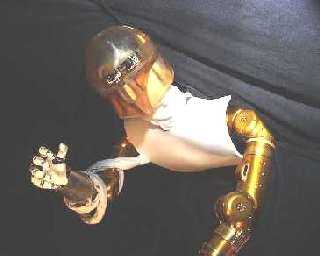
NASA
The
Robonaut hand replicates the size and capability of an astronauts
hand
|
Many ground breaking dexterous robot hands have been developed over
the past two decades. These devices make it possible for a robot
manipulator to grasp and manipulate objects that are not designed
to be robotically compatible. While several grippers have been designed
for space use and some even tested in space, no dexterous robotic
hand has been flown in EVA conditions. The Robonaut Hand is one
of the first under development for space EVA use and the closest
in size and capability to a suited astronaut’s hand. Robonaut Hand
will be able to fit into all the required places. Joint travel for
the wrist pitch and yaw is designed to meet or exceed the human
hand in a pressurized glove. The hand and wrist parts are sized
to reproduce the necessary strength to meet maximum EVA crew requirements.
EVA space compatibility separates the Robonaut Hand from many others.
All component materials meet outgassing restrictions to prevent
contamination that could interfere with other space systems. Parts
made of different materials are toleranced to perform acceptably
under the extreme temperature variations experienced in EVA conditions.
Brushless motors are used to ensure long life in a vacuum. All parts
are designed to use proven space lubricants. The Robonaut Hand has
a total of fourteen degrees of freedom. It consists of a forearm
which houses the motors and drive electronics, a two degree of freedom
wrist, and a five finger, twelve degree of freedom hand. The forearm,
which measures four inches in diameter at its base and is approximately
eight inches long, houses all fourteen motors, 12 separate circuit
boards, and all of the wiring for the hand. The hand itself is broken
down into two sections : a dexterous work set which is used for
manipulation, and a grasping set which allows the hand to maintain
a stable grasp while manipulating or actuating a given object. This
is an essential feature for tool use. The dexterous set consists
of two three degree of freedom fingers (pointer and index) and a
three degree of freedom opposable thumb. The grasping set consists
of two, one degree of freedom fingers (ring and pinkie) and a palm
degree of freedom. All of the fingers are shock mounted into the
palm.

NASA
The
range of motion in the arm exceeds that of a human
|
Arms
Robonaut's arms
are human scale manipulators designed to fit within the exterior
volume of an Astronaut's suit (the EMU). Beyond its volume, design
objectives were human equivalent strength, human scale reach, thermal
endurance to match an 8 hour EVA, fine motion, high bandwidth dynamic
response, redundancy, safety, and a range of motion that exceeds
that of a human limb. The arm has a dense packaging of joints and
avionics developed with the mechatronics philosophy. The endoskeletal
design of the arm houses thermal vacuum rated motors, harmonic drives,
fail-safe brakes and 16 sensors in each joint (saves on the health
insurance quotes!). Custom lubricants, strain gages, encoders
and absolute angular position sensors were developed in house to
make the dense packaging possible. The Roll-Pitch-Roll-Pitch-Roll-Pitch-Yaw
kinematic tree is covered in a series of synthetic fabric layers,
forming a skin that provides protection from contact and extreme
thermal variations in the environment of space. Two of these arm
joints have already been tested in a thermal vacuum chamber at JSC,
where they performed well as the temperature was varied from -25C
to 105C.
Robonaut
Control System
The Robonaut control system architecture must respond to several
interesting challenges. It must provide safe, reliable control for
47+ degrees of freedom. It must be controllable via direct teleoperation,
shared control, and full autonomy. It must maintain performance
in a harsh thermal environment. It must execute at the required
rate on reasonable computing hardware. These challenges cannot be
met by using only classical robot control methods. Advanced control
theory in the areas of grasping, force control, intelligent control,
and shared control must be developed to the point where the control
is suitable for critical applications to fully realize the capability
of the Robonaut. The overall control
architecture is being developed around the concept of creating sub-autonomies
which are used to build the main system. These autonomies each combine
controllers, safety systems, low-level intelligence, and sequencing.
As a result, each is a self contained, peer system which interacts
with the other peers.
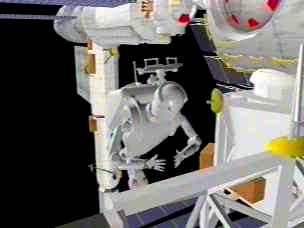
NASA
Space
walks will be the work of robots
|
An example
of the force controller sub-autonomy is shown below. The force safety
system is an integral part of the sub-autonomy. Its limits are controlled
by the force sequencer which configures the sub-autonomy for the
selected force mode. When the safety system detects a problem, an
input reaches a design criteria, or a mode change occurs the force
sequencer handles an orderly configuration change of the force control
sub-autonomy. The mode of the joint control system required to implement
the force mode is decided by the force sequencer and is sent to
the joint control sub-autonomy. System sub-autonomies include task
sequences, Cartesian control, vision, teleoperator interface, joint
control, and grasping among others. Higher level sub-autonomies
make decisions as to what services the lower level sub-autonomies
need to provide
to implement the required tasks. The overall system design makes
conflicts in requests for services either impossible or allows for
arbitration by system level autonomies.
Each sub-autonomy
handles its own internal safety and decision making. If a failure
occurs, a lower level sub-autonomy can request a shutdown or reconfiguration
from a higher level sub-autonomy or the main system controller which
will handle the system level actions required. The advantages of
this approach are each sub-autonomy can be tested individually and
the object orientedness of the system is enhanced. Computing environment
The computing environment chosen for the Robonaut project includes
several state-of-the-art technologies.
The PowerPC
processor was chosen as the real-time computing platform for its
performance and its continued development for space applications.
The computers and their required I/O are connected via a VME backplane.
The processors run the VxWorks real-time operating system. This
combination of flexible computing hardware and operating system
supports varied development activities. The software for Robonaut
is written in C and C++. ControlShell, a software development environment
for object oriented, real-time software development, is used extensively
to aid in the development process. ControlShell provides a graphical
development environment which enhances the understanding of the
system and code reusability.
|