Looking at the development of a variety of
new safe and fast technologies to propel explorers across the solar
system.
by Trudy E. Bell
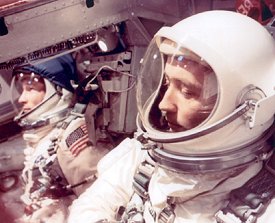
more
Astronauts
Edward White (left) and James McDivitt wait for liftoff
inside their Gemini IV spacecraft.
|
"Mum, are we there yet?"
Every parent has heard that cry from
the back seat of the car. It usually begins about 15 minutes after
the start of any family trip. Good thing we rarely travel more than
a few hundred or a few thousand miles from home.
But what if you were travelling to,
say, Mars? Even at its closest approach to Earth every couple of
years, the red planet is always at least 35 million miles away.
Six months there and six months back - at best.
"Chemical rockets are just too slow,"
laments Les Johnson, manager for in-space transportation technologies
at NASA's Marshall Space Flight Center. "They burn all their propellant
at the beginning of a flight and then the spacecraft just coasts
the rest of the way." Although spacecraft can be sped up by gravity
assist - a celestial crack-the-whip around planets, such as the one
around Saturn that flung Voyager 1 to the edge of the solar system - round-trip
travel times between planets are still measured in years to decades.
And a journey to the nearest star would take centuries if not millennia.
Worse yet, chemical rockets are just
too fuel-inefficient. Think of driving in a gas guzzler across a
country with no gas stations. You'd have to carry boatloads of gas
and not much else. In space missions, what you can carry on your
trip that isn't fuel (or tanks for fuel) is called the payload mass - e.g.,
people, sensors, samplers, communications gear and food. Just as
gas mileage is a useful figure of merit for the fuel efficiency
of a car, the "payload mass fraction" - the ratio of a mission's
payload mass to its total mass - is a useful figure of merit for
the efficiency of propulsion systems.
With today's chemical rockets, payload
mass fraction is low. "Even using a minimum-energy trajectory to
send a six-person crew from Earth to Mars, with chemical rockets
alone the total launch mass would top 1,000 metric tons - of
which some 90 percent would be fuel," said Bret G. Drake,
manager for space launch analysis and integration at Johnson Space
Center. The fuel alone would weigh twice as much as the completed
International Space Station.
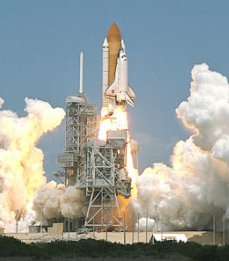
more
When the
space space shuttle lifts off, most of what leaves the launch
pad is chemical fuel. The orange external fuel tank and
the two white solid rocket boosters are discarded before
the shuttle reaches orbit.
|
A single Mars expedition with today's
chemical propulsion technology would require dozens of launches
- most of which would simply be launching chemical fuel. It's as
if your 1-ton compact car needed 9 tons of petrol to drive from
New York City to San Francisco because it averaged only a mile per
gallon.
In other words, low-performance propulsion
systems are one major reason why humans have not yet set foot on
Mars.
More efficient propulsion systems increase
the payload mass fraction by giving better "gas mileage" in space.
Since you don't need as much propellant, you can carry more stuff,
go in a smaller vehicle, and/or get there faster and cheaper. "The
key message is: we need advanced propulsion technologies to enable
a low-cost mission to Mars," Drake says.
Thus, NASA is now developing ion drives,
solar sails, and other exotic propulsion technologies that for decades
have whooshed humans to other planets and stars - but only in the
pages of science fiction.
So, what are the science-fact options?
NASA is hard at work on two basic approaches.
The first is to develop radically new rockets that have an order-of-magnitude
better fuel economy than chemical propulsion. The second is to develop
"propellant-free" systems that are powered by resources abundant
in the vacuum of deep space.
All these technologies share one key
characteristic: they start slowly, like the proverbial tortoise,
but over time turn into a hare that actually wins a race to Mars - or
wherever. They rely on the fact that a small continuous acceleration
over months can ultimately propel a spacecraft far faster than one
enormous initial kick followed by a long period of coasting.
Technically speaking, they're all systems
with low thrust (meaning you would barely feel the oh-so-gentle
acceleration, equivalent to that of the weight of a piece of paper
lying on your palm) but long operating times. After months of continuing
small acceleration, you'd be clipping along at many miles per second!
In contrast, chemical propulsion systems are high thrust and short
operating times. You're crushed back into the seat cushions while
the engines are firing, but only briefly. After that the tank is
empty.
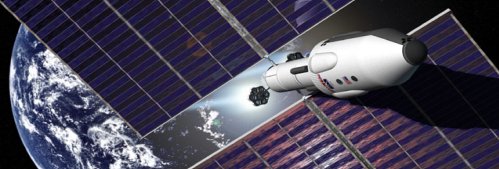
Image Credit: John
Frassanito & Associates, Inc
This low-thrust
spaceship (an artist's concept) is propelled by an ion engine
and powered by solar electricity. Eventually the craft will
pick up speed - a result of relentless acceleration - and
race along at many miles per second.
|
"A rocket is anything that throws something
overboard to propel itself forward," Johnson pointed out. (Don't
believe that definition? Sit on a skateboard with a high-pressure
hose pointed one way, and you will be propelled in the opposite
way).
Leading candidates for the advanced
rocket are variants of ion engines. In current ion engines, the
propellant is a colourless, tasteless, odourless inert gas, such
as xenon. The gas fills a magnet-ringed chamber through which runs
an electron beam. The electrons strike the gaseous atoms, knocking
away an outer electron and turn neutral atoms into positively-charged
ions. Electrified grids with many holes (15,000 in today's versions)
focus the ions toward the spaceship's exhaust. The ions shoot past
the grids at speeds of up to more than 100,000 miles per hour (compare
that to an Indianapolis 500 racecar at 225 mph) - accelerating out
the engine into space, so producing thrust.
Where does the electricity come from
to ionize the gas and charge the engine? Either from solar panels
(so-called solar electric propulsion) or from fission or fusion
(so-called nuclear electric propulsion). Solar electric propulsion
engines would be most effective for robotic missions between the
sun and Mars, and nuclear electric propulsion for robotic missions
beyond Mars where sunlight is weak or for human missions where speed
is of the essence.
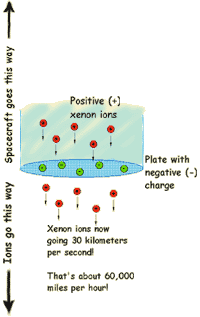
Credit: JPL's Space
Place.
The inner
workings of an ion engine.
|
Ion drives work. They've proven their
mettle not only in tests on Earth, but in working spacecraft - the
best-known being Deep Space 1, a small technology-testing mission
powered by solar electric propulsion that flew by and took pictures
of Comet Borrelly in September, 2001. Ion drives like the one that
propelled Deep Space 1 are about 10 times as efficient as chemical
rockets.
The lowest-mass propulsion systems,
however, may be those that carry no on-board propellant at all.
In fact, they're not even rockets. Instead, in true pioneer style,
they "live off the land" - relying for energy on natural resources
abundant in space, much as pioneers of yore relied for food on trapping
animals and finding roots and berries on the frontier.
The two leading candidates are solar
sails and plasma sails. Although the effect is similar, the operating
mechanisms are very different.
A solar sail consists of an enormous
area of gossamer, highly reflective material that is unfurled in
deep space to capture light from the sun (or from a microwave or
laser beam from Earth). For very ambitious missions, sails could
range up to many square kilometers in area.
Solar sails take advantage of the fact
that solar photons, although having no mass, do have momentum - several
micronewtons (about the weight of a coin) per square meter at the
distance of Earth. This gentle radiation pressure will slowly but
surely accelerate the sail and its payload away from the sun, reaching
speeds of up to 150,000 miles per hour, or more than 40 miles per
second.
A common misconception is that solar
sails catch the solar wind, a stream of energetic electrons and
protons that boil away from the Sun's outer atmosphere. Not so.
Solar sails get their momentum from sunlight itself. It is possible,
however, to tap the momentum of the solar wind using so-called "plasma
sails."
Plasma sails are modelled on Earth's
own magnetic field. Powerful on-board electromagnets would surround
a spacecraft with a magnetic bubble 15 or 20 kilometers across.
High-speed charged particles in the solar wind would push the magnetic
bubble, just as they do Earth's magnetic field. Earth doesn't move
when it's pushed in this way - our planet is too massive. But a
spacecraft would be gradually shoved away from the Sun. (An added
bonus: just as Earth's magnetic field shields our planet from solar
explosions and radiation storms, so would a magnetic plasma sail
protect the occupants of a spacecraft.)
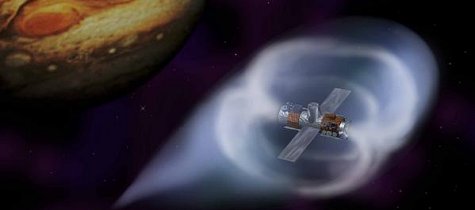
An artist's
concept of a space probe inside a magnetic bubble (or "plasma
sail"). Charged particles in the solar wind hit the bubble,
apply pressure, and propel the spacecraft.
|
Of course, the original, tried-and-true
propellant-free technology is gravity assist. When a spacecraft
swings by a planet, it can steal some of the planet's orbital momentum.
This hardly makes a difference to a massive planet, but it can impressively
boost the velocity of a spacecraft. For example, when Galileo swung
by Earth in 1990, the speed of the spacecraft increased by 11,620
mph; meanwhile Earth slowed down in its orbit by an amount less
than 5 billionths of an inch per year. Such gravity assists are
valuable in supplementing any form of propulsion system.
Okay, now that you've zipping through
interplanetary space, how do you slow down at your destination enough
to go into a parking orbit and prepare for landing? With chemical
propulsion, the usual technique is to fire retrorockets - once again,
requiring large masses of onboard fuel.
A far more economical option is promised
by aerocapture - braking the spacecraft by friction with the destination
planet's own atmosphere. The trick, of course, is not to let a high-speed
interplanetary spacecraft burn up. But NASA scientists feel that,
with an appropriately designed heat shield, it would be possible
for many missions to be captured into orbit around a destination
planet with just one pass through its upper atmosphere.
"No single propulsion technology will
do everything for everybody," Johnson cautioned. Indeed, solar sails
and plasma sails would likely be useful primarily for propelling
cargo rather than humans from Earth to Mars, because "it takes too
long for those technologies to get up to escape velocity," Drake
added.
Nonetheless, a hybrid of several technologies
could prove to be very economical indeed in getting a manned mission
to Mars. In fact, a combination of chemical propulsion, ion propulsion,
and aerocapture could reduce the launch mass of a 6-person Mars
mission to below 450 metric tons (requiring only six launches) - less
than half that attainable with chemical propulsion alone.
Such a hybrid mission might go like
this: Chemical rockets, as usual, would get the spacecraft off the
ground. Once in low-Earth orbit, ion drive modules would ignite,
or ground controllers might deploy a solar or plasma sail. For 6
to 12 months, the spaceship - temporarily unmanned to avoid exposing
the crew to large doses of radiation in Earth's Van Allen radiation
belts - would spiral away, gradually accelerating up to a final high
Earth-departure orbit. The crew would then be ferried out to the
Mars vehicle in a high-speed taxi; a small chemical stage would
then kick the vehicle up to escape velocity, and it would head onward
to Mars.
As Earth and Mars revolve in their
respective orbits, the relative geometry between the two planets
is constantly changing. Although launch opportunities to Mars occur
every 26 months, the optimal alignments for the cheapest, fastest
possible trips happen every 15 years - the next one coming in 2018.
Perhaps by then we'll have a different
answer to the question, "Houston, are we there yet?"
|