A microbe that grows in the Dead Sea
is teaching scientists about the art of DNA repair.
by Patrick L Barry
You can learn a lot
from a microbe. Right now, a tiny critter from the Dead Sea is teaching
scientists new things about biotechnology, cancer, possible life
on other worlds. And that's just for starters:
This microbe, called
Halobacterium, may hold the key to protecting astronauts
from one of the greatest threats they would face during a mission
to Mars: space radiation. The harsh radiation of interplanetary
space can penetrate astronauts' bodies, damaging the DNA in their
cells, which can cause cancer and other illnesses. DNA damage is
also behind cancers that people suffer here on Earth.
Halobacterium
appears to be a master of the complex art of DNA repair. This mastery
is what scientists want to learn from: In recent years, a series
of experiments by NASA-funded researchers at the University of Maryland
has probed the limits of Halobacterium's powers of self-repair,
using cutting-edge genetic techniques to see exactly what molecular
tricks the "master" uses to keep its DNA intact.
"We have completely
fragmented their DNA. I mean we have completely destroyed it by
bombarding it with [radiation]. And they can reassemble their entire
chromosome and put it back into working order within several hours,"
says Adrienne Kish, member of the research group studying Halobacterium
at the University of Maryland.
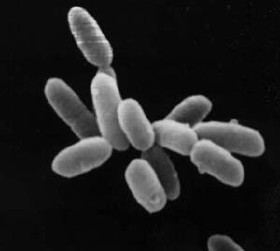
more
Cells of Halobacterium as seen through a high-powered microscope.
The individual cells in this image are about 5 microns
long.
|
Being a virtuoso at
repairing damaged DNA makes Halobacterium one hardy little
microbe: in experiments by the Maryland research group, Halobacterium
has survived normally-lethal doses of ultraviolet radiation (UV),
extreme dryness, and even the vacuum of space.
The Dead Sea is not so dead
But why is Halobacterium such a tenacious survivor? What
caused it to evolve such dexterous DNA repair mechanisms? And how
do those mechanisms work?
Jocelyne DiRuggiero, leader of the Maryland research group, has
been exploring these questions for the last five years. She believes
the answer stems from the fact that Halobacterium naturally
lives in some rather inhospitable places: ultra-salty bodies of
water such as the Dead Sea.
Most sea life would quickly shrivel up and die in the Dead Sea's
briny water, which is 5 to 10 times saltier than normal seawater.
The extreme saltiness damages an organism's cells, and especially
the DNA inside those cells. This happens because DNA molecules are
accustomed to being surrounded by a dense swarm of water molecules,
and the DNA actually depends on the influence of these water molecules
to keep its double-helix structure intact and to avoid damage. But
in ultra-salty waters, the dissolved salt crowds out the water molecules.
Partially deprived of the contact with water they need, the long
strands of DNA suffer damage and even break, causing the cell to
malfunction or die.
Evolving to cope with
a salty lifestyle could explain why Halobacterium is so good
at surviving radiation and other ravages, DiRuggiero reasons:

Credit: Purdue University
The
Dead Sea is 5+ times saltier than Earth's oceans. As water
evaporates, salt is left behind. When the saturation point
is reached, the salt forms these pillars.
|
"High salt concentrations
lead to the same type of lesion in the DNA that does radiation,"
she explains. "So if the organisms are adapted to extreme saltiness,
they have the machinery to repair those lesions when they encounter
radiation."
DiRuggiero and her
research group have begun revealing this DNA-repair machinery in
a recent series of experiments funded by NASA's Exploration Systems
Mission Directorate.
In some experiments, they exposed Halobacterium cells to
beams of intense UV radiation. "We used UV-C at 254 nm, which is
the most lethal UV wavelength," says DiRuggiero. Most microbes,
like E. coli that lives
in the human gut, would have been completely exterminated, yet 80%
of the Halobacterium cells survived. Indeed, they went
on living and reproducing just fine.
In other experiments, the researchers used a vacuum chamber at NASA's
Goddard Space Flight Center to expose cells of Halobacterium
to a space-like vacuum (1 millitorr). Here, living in very salty
water proved to be Halobacterium's saving grace: as the vacuum
caused the water to evaporate away, the salt was left behind, forming
salt crystals. The tiny cells of Halobacterium became trapped
inside these crystals, along with a bit of entrapped water.
"The salt crystal is
like a little house in which the cells are protecting themselves
from additional desiccation," DiRuggiero explains. The cells can
live in a semi-dormant state within the crystals for a long time.
When dissolved back into water, the cells spring to life again,
repair all the damage to their DNA caused by the partial desiccation,
and go right on living.
Some scientists even
claim to have found living cells of Halobacterium encased
in salt deposits that are 250 million years old. (see journal references
below) The claim is controversial, but if true, it could have some
profound implications for the hunt for microbial life on Mars. Evidence
from the Mars Exploration Rovers, Spirit and Opportunity, announced
in March suggests that the Martian surface once had pools of salty
water, which slowly evaporated away.

Image credit: Albert Lau
A repair enzyme correcting an error in a DNA molecule. The
enzyme is on the right in orange and green, and part of
the double-helix-shaped DNA is on the left in blue.
|
"So if microbial life
evolved on Mars and then the water evaporated, and if the microbes
are trapped in salt crystals, they could still be there, and still
viable. Given the data that we have from Earth, that's entirely
possible," Kish says.
Reading
the "book of life"
To understand how these cells of Halobacterium managed to
survive in their experiments, DiRuggiero's team sent the "victims"
of their tests to the Institute for Systems Biology in Seattle.
There, scientists used a modern genetics tool called a "DNA microarray"
to see a complete picture of Halobacterium's response to
being damaged: the full set of molecular tools that spring into
action in the wake of a UV dose or exposure to space-like vacuum.
These "molecular repair tools" belong to a category of proteins
called enzymes. Enzymes are the workhorses of all living cells:
they catalyze the thousands of chemical reactions necessary for
life, such as breaking down food or repairing flaws in DNA. Halobacterium always keeps a certain amount
of repair enzymes on hand, so when a radiation dose occurs, this
stash of enzymes can quickly administer "first aid" to the DNA.
But then it must also ramp up production of other repair enzymes
to continue the repair, activating the genes that produce those
enzymes. It's that boost in gene activity that the microarray tests
can detect, thus showing which enzymes are important for Halobacterium's remarkable DNA-repair
abilities.

Image credit: James Smiley
A DNA
microarray, as seen through a microscope. Each tiny dot
corresponds to one of the organism's thousands of genes,
and the color of the dot indicates the activity level
of that gene.
|
From those microarrays,
DiRuggiero's team has learned that when it comes to DNA repair,
Halobacterium is something of a "Renaissance bug." It dabbles
in a bit of everything. Its genome of only 2,400 genes contains
several distinct sets of DNA-repair mechanisms. Some of these sets
of tools are like the DNA-repair tools found in plants and animals,
other sets are more like those of bacteria, and still others are
characteristic of a lesser-known group of life called "Archaea"
(the group that Halobacterium belongs to). Halobacterium
has them all. Beyond even that, Halobacterium has a few novel
DNA-repair mechanisms that no one has ever seen before!
Learning how all these repair mechanisms work could teach scientists
a lot about how DNA repair occurs in humans, and perhaps point to
ways to enhance people's natural ability to cope with damage to
their DNA - a possible boon to astronauts.
"Many of the repair
proteins in the Archaea are very similar to that of Eukarya - [the
group of life that includes] you and me - and therefore Archaea
can be used as a simple model system to study the more complex processes
that occur in eukaryotes," DiRuggiero explains.
Some of these novel molecular tools could also prove to be useful
for industry and biotechnology, DiRuggiero suspects. After all,
it was in studying a cousin of Halobacterium - a heat-loving
microbe - that scientists found the DNA-copying protein that made
it possible to sequence entire genomes. The Human Genome Project
would have never happened without it.
Not bad for a humble microbe.
|