Driving, digging, mining: these are
things astronauts will be doing one day in the sands of Mars. It's
not as simple as it sounds.
by Trudy E. Bell and Dr. Tony
Phillips
Imagine this scenario.
The year is 2030 or thereabouts. After voyaging six months from
Earth, you and several other astronauts are the first humans on
Mars. You're standing on an alien world, dusty red dirt beneath
your feet, looking around at a bunch of mining equipment deposited
by previous robotic landers.
Echoing in your ears
are the final words from mission control: "Your mission, should
you care to accept it, is to return to Earth - if possible using
fuel and oxygen you mine from the sands of Mars. Good luck!"
It sounds simple enough,
mining raw materials from a rocky, sandy planet. We do it here on
Earth, why not on Mars, too? But it's not as simple as it sounds.
Nothing about granular physics ever is.
Granular physics is
the science of grains, everything from kernels of corn to grains
of sand to grounds of coffee. These are common everyday substances,
but they can be vexingly difficult to predict. One moment they behave
like solids, the next like liquids. Consider a dump truck full of
gravel. When the truck begins to tilt, the gravel remains in a solid
pile, until at a certain angle it suddenly becomes a thundering
river of rock.
Understanding granular
physics is essential for designing industrial machinery to handle
vast quantities of small solids - like fine Martian sand.
The problem is, even
here on Earth "industrial plants don't work very well because
we don't understand equations for granular materials as well as
we understand the equations for liquids and gases," says James
T. Jenkins, professor of theoretical and applied mechanics at Cornell
University in Ithaca, N.Y. "That's why coal-fired power plants
operate at low efficiencies and have higher failure rates compared
to liquid-fuel or gas-fired power plants."
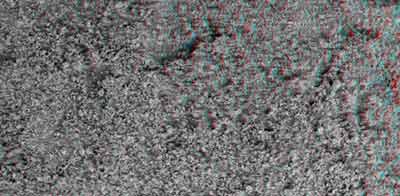
Mars
soil in 3D, photographed by the Spirit rover in 2004.
Put on your red-blue glasses and take a look.
|
So "do we understand
granular processing well enough to do it on Mars?" he asks.
Let's start with excavation:
"If you dig a trench on Mars, how steep can the sides be and
remain stable without caving in?" wonders Stein Sture, professor
of civil, environmental, and architectural engineering and associate
dean at the University of Colorado in Boulder. There's no definite
answer, not yet. The layering of dusty soils and rock on Mars isn't
well enough known.
Some information about
the mechanical composition of the top meter or so of Martian soils
could be gained by ground-penetrating radar or other sounding devices,
Sture points out, but much deeper and you "probably need to
take core samples." NASA's Phoenix Mars lander (landing 2008)
will be able to dig trenches about a half-meter deep; the 2009 Mars
Science Laboratory will be able to cut out rock cores. Both missions
will provide valuable new data.
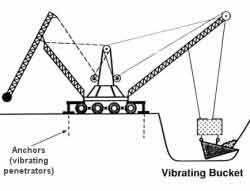
Credit:
Stein Sture
Mars-cranes
might use vibrating buckets for excavation.
|
To go even deeper,
Sture (in connection with the University of Colorado's Center for
Space Construction) is developing innovative diggers whose business
ends vibrate into soils. Agitation helps break cohesive bonds holding
compacted soils together and can also help mitigate the risk of
soils collapsing. Machines like these might one day go to Mars,
too.
Another problem is
"hoppers" - the funnels miners use to guide sand and gravel
onto conveyor belts for processing. Knowledge of Martian soils would
be vital in designing the most efficient and maintenance-free hoppers.
"We don't understand why hoppers jam," Jenkins says. Jams
are so frequent, in fact, that "on Earth, every hopper has
a hammer close by." Banging on the hopper frees the jam. On
Mars, where there would be only a few people around to tend equipment,
you'd want hoppers to work better than that. Jenkins and colleagues
are researching why granular flows jam.
And then there's transportation:
The Mars rovers Spirit and Opportunity have had little trouble driving
miles around their landing sites since 2004. But these rovers are
only about the size of an average office desk and only about as
massive as an adult. They're go-carts compared to the massive vehicles
possibly needed for transporting tons of Martian sand and rock.
Bigger vehicles are going to have a tougher time getting around.
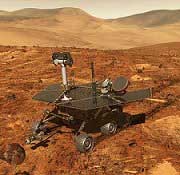
Mars
rover Spirit, an artist's rendition. Spirit and her twin
Opportunity have been roaming Mars since January 2004.
|
Sture explains: As
early as the 1960s when scientists were first studying possible
solar-powered rovers for negotiating loose sands on the Moon and
other planets, they calculated "that the maximum viable continuous
pressure for rolling contact pressure over Martian soils is only
0.2 pounds per square inch (psi)," especially when traveling
up or down slopes. This low figure has been confirmed by the behaviour
of Spirit and Opportunity.
A rolling contact pressure
of only 0.2 psi "means that a vehicle has to be light-weight
or has to have a way of effectively distributing the load to many
wheels or tracks. Reducing contact pressure is crucial so the wheels
don't dig into soft soil or break through duricrusts [thin sheets
of cemented soils, like the thin crust on windblown snow on Earth]
and get stuck."
That requirement implies
that a vehicle for moving heavier loads - people, habitats, equipment - might
be "a huge Fellini-type thing with wheels 4 to 6 meters (12
to 18 feet) in diameter," says Sture, referring to the famous
Italian director of surreal films. Or it might have enormous open-mesh
metal treads like a cross between highway-construction backhoes
on Earth and the lunar rover used during the Apollo program on the
Moon. Thus, tracked or belted vehicles seem promising for carrying
large payloads.
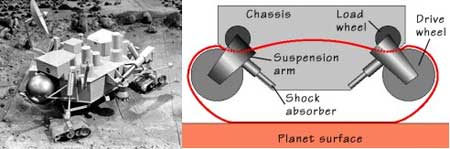
Photo credit: Stein Sture
An
experimental Elastic Loop Mobility System that might work
on worlds with dusty soil like Mars and the Moon.
|
A final challenge facing
granular physicists is to figure out how to keep equipment operating
through Mars' seasonal dust storms. Martian storms whip fine dust
through the air at velocities of 50 m/s (100+ mph), scouring every
exposed surface, sifting into every crevice, burying exposed structures
both natural and manmade, and reducing visibility to meters or less.
Jenkins and other investigators are studying the physics of aeolian
[wind] transporting of sand and dust on Earth, both to understand
the formation and moving of dunes on Mars, and also to ascertain
what sites for eventual habitats might be best protected from prevailing
winds (for example, in the lee of large rocks).
Returning to Jenkins's
big question, "do we understand granular processing well enough
to do it on Mars?" The unsettling answer is: we don't yet know.
Working with imperfect
knowledge is okay on Earth because, usually, no one suffers much
from that ignorance. But on Mars, ignorance could mean reduced efficiency
or worse preventing the astronauts from mining enough oxygen and
hydrogen to breathe or use for fuel to return to Earth.
Granular physicists
analyzing data from the Mars rovers, building new digging machines,
tinkering with equations, are doing their level best to find the
answers. It's all part of NASA's strategy to learn how to get to
Mars ... and back again.
|