Deep within the cells we are made of, squishy skeletons feel
the effects of gravity... and respond in unexpected ways.
by
Karen Miller and Dr Tony Phillips
Sculptor Kenneth Snelson's "Needle
Tower" is a fragile-looking thing. Criss-crossing rods suspended
by taut wires soar perilously upward 20 meters high. Surely it ought
to crumble or fall over? Yet it doesn't. When the wind blows, the
Needle Tower bends, not breaks. When someone shoves it, it shoves
back. The tower is lightweight, strong and curiously beautiful.
Just
like the skeletons of cells. That's right,
cells have skeletons. They're not made of calcium like the bones that rattle on
Halloween. Cell skeletons-biologists call them cytoskeletons - consisting of protein
molecules arranged into chains. Cytoskeletons give cells their shape, help cells
move, and hold the nucleus in place.
Like Snelson's sculptures, cytoskeletons have 'tensegrity' - short for
tensional integrity. They balance compression with tension, and yield to forces
without breaking. In the Needle Tower, the wires carry tension and the rods bear
compression. In a cytoskeleton, protein chains - some thin, some thick and some
hollow, take the place of wires and rods. Linked together they form a stable,
but flexible, structure.
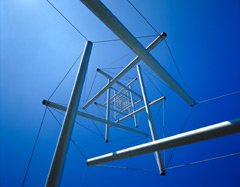
more
The Needle
Tower - a 1969 tensegrity sculpture by artist Kenneth Snelson
- viewed from below.
|
NASA is interested in cytoskeletons
because cytoskeletons respond to gravity. Weight can provide both
tension and compression. But what happens (during space travel,
for example) when weight vanishes? Do cells behave differently when
their cytoskeletons relax?
Harvard
cell biologist Don Ingber is a leader among researchers who have been working
to find out. "The cytoskeleton perceives
gravity-or any force- through special proteins known as integrins, which poke
through the cell's surface membrane," explains Ingber. Inside the cell, they're
hooked to the cytoskeleton. Outside, they latch onto a framework known as the
extracellular matrix - a fibrous scaffolding to which cells are anchored in our
bodies. Ingber and his colleagues have
shown that when integrins move, the cytoskeleton stiffens. They did it by coating
small magnetic beads, about 1 to 10 microns in size, with special molecules that
bind to integrins. They attached the beads to the integrins and then applied a
magnetic field. "The beads turned and
tried to align with the field, just like a compass needle would want to align
with the earth's magnetic field," explains Ingber. The beads twisted the integrins
and, in turn, tweaked the cytoskeleton. As more stress was applied, the cytoskeleton
became stiffer and stiffer. In fact, it become so stiff that the beads couldn't
be turned much past a few degrees!

more
Cytoskeletons
of human endothelial cells glow green in this immunofluorescent micrograph. The
filaments meet in triangular structures resembling a geodesic dome - an example
of tensegrity. | Tugging
on integrins not only caused the cytoskeleton to stiffen, it also activated certain
genes. "Activating a gene" means coaxing a gene to generate RNA and proteins.
That's important because proteins are little messages that signal the cell to
take action. Tickling the cytoskeleton, it seems, can make cells switch between
different genetic programs.
Even before
the magnetic bead experiment, Ingber's group at Harvard had already
discovered a link between cell geometry and cell behaviour. In one
experiment they forced living cells to take on different shapes -
spherical or flattened, square or round - by placing them on tiny
adhesive islands of extracellular matrix. Cells that were flat and
stretched tended to divide. Cells that were round and cramped tended
to die.
Says
Ingber: "Mechanical restructuring of the cell and cytoskeleton apparently tells
the cell what to do." Very
flat cells with taut cytoskeletons somehow sense that more cells are needed-to
cover a cut, for example. Rounder, cramped cells might sense an overpopulation
problem and decide it's time to die and make room for others. In either case,
they are responding to a control system in which the shape-shifting cytoskeleton
serves as a switching mechanism. The
potential implications of this research are vast - and not limited to space travel.
It has already led to a prospective cancer treatment based on changes in cell
shape. And it could provide new treatments for osteoporosis, cardiac disease,
lung problems and developmental abnormalities. Every tissue in the body, says
Ingber, has some disease that results from cells responding abnormally to mechanical
forces. "By
pursuing the question of how cells sense gravity we've uncovered entirely new
aspects of cell regulation."

more
Cytoskeletons
give red blood cells their characteristic flat shape.
|
Ingber believes that tensegrity is
a core organising principle of the entire physical world. Self-stabilizing
structures form spontaneously at every scale - cytoskeletons are
merely one example. Another would be spherical carbon molecules
called "BuckyBalls" that look like atomic soccer balls. Clay molecules
also arrange themselves into tensegrity patterns that some researchers
think harboured the first microscopic life forms on Earth. Even
the universe itself, with its black holes (compression) and gravitationally
linked galaxies (tension), may be a tensegrity structure.
"I
gave a talk once at NASA on evolutionary biology," he recalls. "The last slide
of my talk was a picture of the universe: super clusters of galaxies. Next to
it was a one of capillary cells in a dish, formed into networks. The two pictures
looked identical."
|