Tomorrow's spacecraft will be built using
advanced materials with mind-boggling properties.
by Patrick L. Barry
"What I'm really looking for," you say
to the salesman, "is a car that goes at least 10,000 miles between
fill-ups, repairs itself automatically, cruises at 500 mph, and weighs
only a few hundred pounds."
As he stands there wide-eyed, you add, "Oh yeah, and I can only
spend about a quarter of what these other cars cost."
A request like this is sure to get you laughed off the new-car
lot. But in many ways, this dream car is a metaphor for the space
vehicles we'll need to expand our exploration of the solar system
in the decades to come. These new spacecraft will need to be faster,
lighter, cheaper, more reliable, more durable, and more versatile,
all at the same time.
Impossible? Before you answer, consider how a rancher from 200 years
ago might have reacted if a man had asked to buy a horse that could
run 100 mph for hours on end, carry his entire family and all their
luggage, and sing his favourite songs to him all the while! Today
we call them minivans.
Revolutions in technology - like the Industrial Revolution that
replaced horses with cars - can make what seems impossible today
commonplace tomorrow.
Such a revolution is happening right now. Three of the fastest-growing
sciences of our day - biotech, nanotech, and information technology - are
converging to give scientists unprecedented control of matter on
the molecular scale. Emerging from this intellectual gold-rush is
a new class of materials with astounding properties that sound more
at home in a science fiction novel than on the laboratory workbench.
Imagine, for example, a substance with 100 times the strength of
steel, yet only 1/6 the weight; materials that instantly heal themselves
when punctured; surfaces that can "feel" the forces pressing on
them; wires and electronics as tiny as molecules; structural materials
that also generate and store electricity; and liquids that can instantly
switch to solid and back again at will. All of these materials exist
today ... and more are on the way.
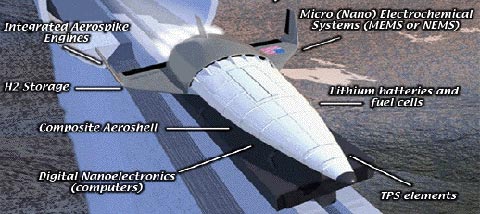
more
A
next-generation minivan? Advanced materials will be essential
for making dramatically improved spacecraft possible.
|
With such mind-boggling materials at hand, building
the better spacecraft starts to look not so far fetched after all.
The challenge of the next-generation
spacecraft hinges on a few primary issues. First and foremost, of
course, is cost.
"Even if all the technical obstacles were solved
today, exploring our solar system still needs to be affordable to
be practical," says Dr. Neville Marzwell, manager of Revolutionary
Aerospace Technology for NASA's Next Decadal Planning Team.
Lowering the cost of space flight primarily means reducing weight.
Each pound trimmed is a pound that won't need propulsion to escape
from Earth's gravity. Lighter spaceships can have smaller, more
efficient engines and less fuel. This, in turn, saves more weight,
thus creating a beneficial spiral of weight savings and cost reduction.
The challenge is to trim weight while increasing
safety, reliability, and functionality. Just leaving parts out won't
do.
Scientists are exploring a range of new technologies that could
help spacecraft slim down. For example, gossamer materials - which
are ultra-thin films - might be used for antennas or photovoltaic
panels in place of the bulkier components used today, or even for
vast solar sails that provide propulsion while massing only 4 to
6 grams per square meter.
Composite materials, like those used in carbon-fiber tennis rackets
and golf clubs, have already done much to help bring weight down
in aerospace designs without compromising strength. But a new form
of carbon called a "carbon nanotube" holds the promise of a dramatic
improvement over composites: The best composites have 3 or 4 times
the strength of steel by weight - for nanotubes, it's 600 times!
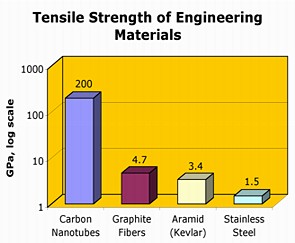
more
The tensile
strength of carbon nanotubes greatly exceeds that of other
high-strength materials. Note that each increment on the
vertical axis is a power of 10.
|
"This phenomenal strength comes from the
molecular structure of nanotubes," explains Dennis Bushnell, a chief
scientist at Langley Research Centre (LaRC), NASA's Centre of Excellence
for Structures and Materials. They look a bit like chicken-wire rolled
into a cylinder with carbon atoms sitting at each of the hexagons'
corners. Typically nanotubes are about 1.2 to 1.4 nanometers across
(a nanometer is one-billionth of a meter), which is only about 10
times the radius of the carbon atoms themselves.
Nanotubes were only discovered in 1991, but already the intense
interest in the scientific community has advanced our ability to
create and use nanotubes tremendously. Only 2 to 3 years ago, the
longest nanotubes that had been made were about 1000 nanometers
long (1 micron). Today, scientists are able to grow tubes as long
as 200 million nanometers (20 cm). Bushnell notes that there are
at least 56 labs around the world working to mass produce these
tiny tubes.
"Great strides are being made, so making bulk materials using nanotubes
will probably happen," Bushnell says. "What we don't know is how
much of this 600 times the strength of steel by weight will be manifest
in a bulk material. Still, nanotubes are our best bet."
Beyond merely being strong, nanotubes will likely be important
for another part of the spacecraft weight-loss plan: materials that
can serve more than just one function.
"We used to build structures that were just dumb, dead-weight holders
for active parts, such as sensors, processors, and instruments,"
Marzwell explains. "Now we don't need that. The holder can be an
integral, active part of the system."
Imagine that the body of a spacecraft could also store power, removing
the need for heavy batteries. Or that surfaces could bend themselves,
doing away with separate actuators. Or that circuitry could be embedded
directly into the body of the spacecraft. When materials can be
designed on the molecular scale such holistic structures become
possible.
Humans can feel even the slightest pinprick anywhere on their bodies.
It's an amazing bit of self-monitoring - possible because your skin
contains millions of microscopic nerve endings as well as nerves
to carry those signals to your brain.
Likewise, materials that make up critical systems in a spaceship
could be embedded with nanometer-scale sensors that constantly monitor
the materials' condition. If some part is starting to fail - that
is, it "feels bad" - these sensors could alert the central computer
before tragedy strikes.
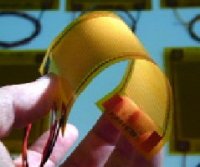
Image courtesy NASA's Morphing Project at LaRC.
This piezoelectric
material, developed at NASA's Langley Research Centre (LaRC),
can "feel" deformations such as bending or surface pressure,
producing a small voltage in response that can act as a
signal for a central computer.
|
Molecular wires could carry the signals from
all of these in-woven sensors to the central computer, avoiding
the impractical bulk of millions and millions of today's wires.
Again, nanotubes may be able to serve this role. Conveniently, nanotubes
can act as either conductors or semi-conductors, depending on how
they're made. Scientists have made molecular wires of other elongated
molecules, some of which even naturally self-assemble into useful
configurations.
Your skin is also able to heal itself. Believe
it or not, some advanced materials can do the same thing. Self-healing
materials made of long-chain molecules called ionomers react to
a penetrating object such as a bullet by closing behind it. Spaceships
could use such skins because space is full of tiny projectiles - fast-moving
bits of debris from comets and asteroids. Should one of these sand-
to pebble-sized objects puncture the ship's armor, a layer of self-healing
material would keep the cabin airtight.
Meteoroids aren't the only hazard; space is filled with radiation,
too. Spaceships in low-Earth orbit are substantially protected by
our planet's magnetic field, which forms a safe bubble about 50,000
km wide centred on Earth. Beyond that distance, however, solar flares
and cosmic rays pose a threat to space travelers.
Scientists are still searching for
a good solution. The trick is to provide adequate shielding without
adding lots of extra weight to the spacecraft. Some lightweight
radiation-shielding materials are currently being tested in an experiment
called MISSE onboard the International Space Station. But these alone won't be
enough.
The real bad guy is Galactic Cosmic Radiation
(GCR) produced in distant supernova explosions. It consists, in
part, of very heavy positive ions - such as iron nuclei - zipping
along at great speed. The combination of high mass and high speed
makes these little atomic "cannon balls" very destructive. When
they pierce through the cells in people's bodies, they can smash
apart DNA, leading to illness and even cancer.
"It turns out that the worst materials you can use for shielding
against GCR are metals," Bushnell notes. When a galactic comic ray
hits a metallic atom, it can shatter the atom's nucleus - a process
akin to the fission that occurs in nuclear power plants. The secondary
radiation produced by these collisions can be worse than the GCR
that the metal was meant to shield.
Ironically, light elements like hydrogen
and helium are the best defence against these GCR brutes, because
collisions with them produce little secondary radiation. Some people
have suggested surrounding the living quarters of the ship with
a tank of liquid hydrogen. According to Bushnell, a layer of liquid
hydrogen 50 to 100 cm thick would provide adequate shielding. But
the tank and the cryogenic system is likely to be heavy and awkward.
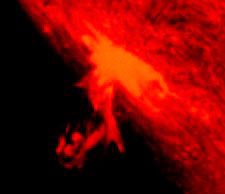
A solar
flare blasts energetic radiation into space
|
Here again, nanotubes might be useful. A lattice
of carbon nanotubes can store hydrogen at high densities, and without
the need for extreme cold. So if our spacecraft of the future already
uses nanotubes as an ultra-lightweight structural material, could
those tubes also be loaded up with hydrogen to serve as radiation
shielding? Scientists are looking into the possibility.
Going one step further, layers of this
structural material could be laced with atoms of other elements
that are good at filtering out other forms of radiation: boron and
lithium to handle the neutrons, and aluminium to sop up electrons,
for example.
Earth's surface is mostly safe from cosmic radiation,
but other planets are not so lucky. Mars, for example, doesn't have
a strong global magnetic field to deflect radiation particles, and
its atmospheric blanket is 140 times thinner than Earth's. These
two differences make the radiation dose on the Martian surface about
one-third as intense as in unprotected open space. Future Mars explorers
will need radiation shielding.
"We can't take most of the materials with us
for a long-term shelter because of the weight consideration. So
one thing we're working on is how to make radiation-shielding materials
from the elements that we find there," says Sheila Thibeault, a
scientist at LaRC who specializes in radiation shielding.
One possible solution is "Mars bricks." Thibeault
explains: "Astronauts could produce radiation-resistant bricks from
materials available locally on Mars, and use them to build shelters."
They might, for example, combine the sand-like "regolith" that covers
the Martian surface with a polymer made on-site from carbon dioxide
and water, both abundant on the red planet. Zapping this mixture
with microwaves creates plastic-looking bricks that double as good
radiation shielding.
"By using microwaves, we can make these bricks quickly using very
little energy or equipment," she explains. "And the polymer we would
use adds to the radiation-shielding properties of the regolith."
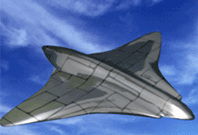
Crafted
from smart materials, tomorrow's airplanes could have self-bending
wings that operate without flaps - thus reducing drag and
lowering fuel costs
|
Mars shelters would need the reliability of
self-sensing materials, the durability of self-healing materials,
and the weight savings of multi-functional materials. In other words,
a house on Mars and a good spacecraft need many of the same things.
All of these are being considered by researchers, Thibeault says.
Mind-boggling advanced materials will come in
handy on Earth, too.
"NASA's research is certainly focused
on aerospace vehicles," notes Anna McGowan, manager of NASA's Morphing
Project (an advanced materials research effort at the Langley Research
Centre). "However, the basic science could be used in many other
areas. There could be millions of spin-offs."
But not yet. Most advanced materials lack the
engineering refinement needed for a polished, robust product. They're
not ready for primetime. Even so, say researchers, it's only a matter
of time: Eventually that car salesman will stop laughing
... and start selling your space-age dream machine.
|