Extinction has always been a controversial subject. Even after
decades of intense study, researchers are still arguing over the
cause of the best-known mass extinction, the death of the
dinosaurs. And theres a topical slant in the idea that
- even today - species are regarded as "endangered" if they are
relatively susceptible to extinction. Then too, what thinking person
could have avoided wondering whether our own species is slated for
extinction and, if so, how long we have before we meet our fate?
The disappearing Mammoth
Two centuries ago extinction held centre-stage in an even more
fundamental debate: whether the Bible should be interpreted literally.
The bones of a very large fossil elephant (a Mammoth)
had been collected in the then new world of North America. Discussing
the Mammoth at the National Institute of Sciences and Arts in Paris
in 1796, the French anatomist Baron Georges Cuvier argued that the
bones belonged to a unique species of which no living examples were
known. It was extremely unlikely that such a large animal could
have gone undetected if its descendants had survived to the present
day, so Cuvier pronounced the species extinct.
This conclusion contradicted religious dogma of the time. A benevolent
creator, it was assumed, would not allow any of his creation to
disappear from the Earth. In this way, Cuvier fired off one of the
first salvos in a debate between theologians and natural historians
that culminated 60 years later with the writings of Charles Darwin
- and still continues in some quarters of society to this day.
Observing extinctions
Since Cuvier's time, extinctions have studied primarily by palaeontologists
- scientists who investigate the remains of ancient life. For all
that is said in popular books, articles and television programmes,
the study of extinction is a surprisingly problematic and young
field of inquiry. Extinction is one of the most common observations
in palaeontology, but it remains very difficult to understand precisely
why a particular ancient plant or animal species went extinct.
Much of this difficulty stems from the way that scientists observe
an extinction. Stated rather badly, we study extinction by observing
what isnt there. When we collect a fossil from a rock deposit,
we assume that the species represented by this fossil lived at the
place and time the rock was formed. If we sample a younger deposit
and find that the species is not present, and if it never reappears
in still younger deposits, we assume the species has become extinct,
and that the time of extinction corresponds to the date of the youngest
observed fossils.
But there may be other explanations for the absence of the species
in younger deposits. For example, the species may have become extinct
in this particular region, but survived to later times in other
areas. Or the younger deposits may record an environment in which
the species never occurred in the first place. A third possibility
is that natural selection changed the appearance of the species
to such an extent that palaeontologists mistakenly assign younger
members to a different species.
A chart of extinctions
These problems mean that we are only just beginning to compile
reliable data on how extinctions have varied over time. And such
data is essential if we want to test different hypotheses on what
has caused extinctions.
Click the
image to see a larger version
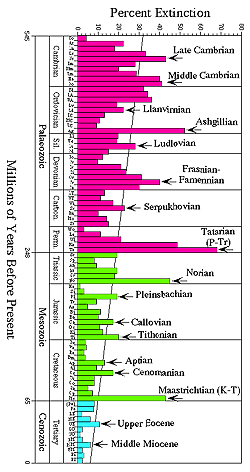
Figure 1. Extinctions over the past 600 million years - mass
extinctions show up as peaks superimposed on a general decline
in extinctions (diagonal line). The mass extinction marked as
'Maastrichtian' was the death of the dinosaurs. |
Reference:
Sepkoski, JJ, Jr. 1994. Extinction and the fossil record. Geotimes
March 15 - 17 |
Figure 1 shows one of the latest summaries of extinction data.
It concentrates on invertebrates - such as snails, clams and coral
species - that lived in ancient oceans. The graph shows the number
of extinctions that occurred within successive small subdivisions
of geological time - called stages - which are typically a few million
years in duration.
There are two particularly noteworthy features. First, there is
a general decline in the number of extinctions over the last 600
million years. Secondly, there are several major peaks
of extinction, which are roughly evenly spaced. Both of these features
are spurring a vigorous debate among palaeontologists.
Extinctions on the decline
The overall trend in Figure 1 shows the number of extinctions
decreasing with time. At face value, it means that a species existing
in the past was more likely to be wiped out than a species existing
now. Is this really the case, and - if so - why?
There are currently three explanations.
The first suggests that the effect isnt real. It occurs
because palaeontologists are more familiar with modern marine organisms
than with species from the remote past. When dealing with recent
fossils, palaeontologists can be fairly confident that they are
classifying them correctly into different species and groups of
species (genera). When they look back to more ancient and unfamiliar
fossils, they are more likely to be fooled into separating them
out into many more genera. So theres a higher chance that
one of these - artificially small - ancient genera would become
extinct.
The second invokes marine organisms moving from the general ocean
into marginal environments, for example around the edges
of continents. Here, they might be sheltered from environmental
changes, and so suffer less extinctions. On the other hand, the
marginal environment might play the opposite role. It could be so
extreme that the species had to evolve into a hardier form, which
was then immune to all but the most profound episodes of global
environmental change.
The third (and most recent) explanation argues that conditions
in the world's oceans have become progressively more favourable
for marine life. In 1994, Prof. Ron Martin of The University of
Delaware summerized geochemical evidence that the oceans themselves
have changed over geological time, by studying isotopes of elements
such as sulphur-34, strontium-87 and carbon-13. These trends match
the declining extinction curve.
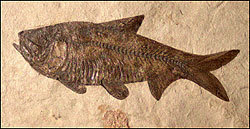 |
photo:
Norman Macleod |
Ocean changes
In Martin's model, the oceans of 600 million years ago were very
different from those of today. Their circulation was sluggish, with
large areas of the ocean floor covered by waters with little or
no dissolved oxygen. They received large amounts of nutrient washed
off from the barren continents (plants and soils had not yet evolved).
And ocean plankton was relatively inefficient at producing organic
materials through photosynthesis.
As time went by, the oceans became more dynamic as plate tectonics
pushed the continents around. Life on land trapped the nutrients
that once ran off into the seas. As a result, the oceans became
host to a greater diversity of plankton. This, in turn, meant they
could support a diverse array of species, increasing the stability
of marine ecosystems - and decreasing the probability of extinction.
Mass extinctions: the three culprits
The second important pattern we can see in Figure 1 are peaks,
where large numbers of species (up to 90%) become extinct within
a short period of time. These are termed mass extinctions. The most
famous - though not the most severe - involved the death of
the dinosaurs about 65 million years ago.
Scientists have proposed many different mechanisms to account
for the pattern of mass extinctions over the last 600 million years.
Currently, the most popular are: a fall in sea level; vast eruptions
of basalt; and the impact of an asteroid or comet.
Interestingly, its not the direct effects that cause the
damage. In all three cases, researchers explain the extinctions
through subsequent drastic changes in the environment. For example,
they may cool the Earth by promoting the formation of clouds, or
lead to global warming as greenhouse gases are released. Nitrogen
and sulphur compounds thrown into the atmosphere may produce acid
rain, and also destroy the ozone layer.
Patterns of extinction
Generally, its difficult link any particular catastrophic
event to a subsequent mass extinction. Instead palaeontologists
comparing the pattern of mass extinctions as a whole with the patterns
of each of the three possible mechanisms. Figure 2 compares the
record of mass extinctions with geological records of sea-level
falls, flood-basalt eruptions, and impacts of bolides (asteroids
or comets).
Click the
image to see a larger version
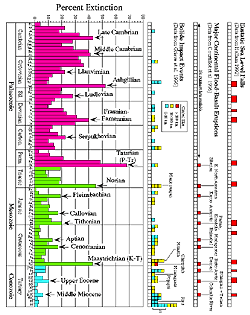
Figure 2. The record of extinctions (bottom), compared with
- from top - major sea-level changes, giant eruptions of basalt,
and impact craters. |
References:
Courtillot, V, Jaeger, J-J, Yang, Z, Féraud, G, Hofmann,
C. 1996. The influence of continental flood basalts on mass
extinctions: where do we stand? In Ryder, G, Fastovsky, D, and
Gartner, S, eds. The Cretaceous-Tertiary event and other catastrophes
in earth history: The Geological Society of America, Special
Paper 307, 513-525. Grieve, R, Rupert, J, Smith, J, Therriault,
A. 1996. The record of terrestrial impact cratering. GSA Today
5: 193-195
Hallam, A. 1992. Phanerozoic sea-level changes. New York; Columbia
University Press. |
Looking at Figure 2, it is clear that impacts show the worst record
of association with mass extinction events. Sizeable impacts are
as likely to have occurred during low-extinction stages as during
high-extinction stages. In fact, theres only one compelling
example of an association between a large impact and a major extinction
event. The Chicxulub event of 65 million years ago was the largest
impact of the last 600 million years, and third largest in the known
history of our planet. It blasted out a large crater in Mexico.
At the same time, we find the Maastrichtian mass extinction event,
also known as the K/T mass extinction. This was the disaster in
which the dinosaurs perished, and it is ranked as the third to fifth
largest extinction event of the last 600 million years.
Moving on to the second possible mechanism, abrupt falls in sea
level show a rather better level of association with extinctions.
Each of the three largest mass extinctions during the last 250 million
years (the time interval for which we have the most accurate time
resolution) corresponds to a major sea-level change. This supports
the idea that rapid falls in sea level have a detrimental effect
on the diversity of marine invertebrates.
Giant eruptions
But the strongest association - by far - comes with the third
prospective extinction mechanism, as seen in the record of continental
flood-basalt eruptions. These are vast outpourings of basaltic lava,
similar to the eruptions responsible for the Hawaiian Island chain,
but taking place entirely on land.
Perhaps the best known is the series of eruptions that took place
on the Indian subcontinent beginning at the very end of the Maastrichtian
stage, 66.5-64.5 million years ago. They poured out over one million
cubic kilometres of lava in just over one million years, forming
the vast Deccan Traps. The eruption did not take place continuously
over the entire million years, but episodically in massive lava
flows that have no counterpart in human history.
Since we have no direct experience of such cataclysmic eruptions,
it is difficult to imagine (much less model) the climatic effects.
Regardless, the close association between the flood-basalt record
and the pattern of extinction events is very difficult to explain
away as mere coincidence. In addition, its worth noting that
the three largest extinctions of the last 250 million years took
place during times of combined sea-level fall and flood-basalt eruption.
Of course, the Maastrichtian event is also associated with the Chicxulub
impact. However, this event is by no means the largest mass extinction
to have occurred and, according to available data on the timing
and magnitudes of impacts, several extinction events of equal and
greater magnitude are not associated with large asteroid impacts.
Weighing up the evidence
As far as any single extinction event is concerned, in all probability
we will never know all the factors involved. Nevertheless, the existing
data provide an insight into the factors that are repeatedly associated
with extinction events in the geological record.
A simple statistical analysis of the data in Figure 2 reveals
the relative strength of the association between each type of catastrophe
and mass extinctions as follows:
- sea-level change 7 out of 14
- continental flood basalt eruptions (over the last 250 million
years) 10 out of 10
- asteroid impact 1 out of 17.
Based on current data, tectonic factors - giving rise to flood-basalt
volcanism and sea-level fall - appear to exhibit the greatest level
of association with large-scale extinction events over the course
of the last 600 million years.
Extraterrestrial impacts - current darlings of the media coverage
of extinctions - have certainly played an important role in Earth
history and may have enhanced the death of the dinosaurs.
However, asteroid impacts do not appear to be the primary agents
responsible for the overall patterns in the geological extinction
record.
Dr Norman MacLeod is an international expert in the
study of extinctions. After a BSc in geology from the University
of Missouri, he received an MSc from the Southern Methodist University
and a PhD from the University of Texas, both in palaeontology. Norm
MacLeod has researched at the University of Michigan and Princeton
University, and is now a member of the Palaeontology Department
at The Natural History Museum, London. As well as extinctions, his
research interests include the quantitative analysis of morphology
and the analysis of evolutionary patterns.
Copyright © FirstScience.com