Scientists continue to ponder one of the Sun's
most closely guarded secrets.
by Dr Tony Phillips
On a cold winter's day a good place
to be is by a roaring fire with a cup of hot cocoa. If the fire
feels a bit too hot - no problem - just move away. As with most
heat sources, the temperature in the vicinity of a fireplace falls
with increasing distance from the hearth.
This simple rule works for fireplaces, but not so well for the atmosphere
of our planet's main source of heating - the Sun. A thermometer
at the at the sun's surface (called the photosphere) would register
a sizzling 6000 degrees C - a bit too warm for comfort. Unlike the
family fireplace, however, moving away from the photosphere doesn't
lower the temperature. In the 3000 km zone between the photosphere
and the corona, the temperature jumps from 6000 C to over one million
degrees. In apparent defiance of common sense, the further away
you go from the Sun's surface into the solar atmosphere, the warmer
the gas becomes!
A 130 year old mystery...
For most of recorded history, the corona
was simply a mysterious pearly white glow around the Sun and Moon
during a total eclipse. It wasn't until the 17th and 18th centuries
that astronomers were certain that the corona surrounded the Sun
and not the Moon. The first hint that the corona might be really
interesting surfaced during the total solar eclipse of August 7,
1869, which was visible from Alaska to North Carolina, when observers
discovered a weak emission line in the green part of the spectrum
of the corona. The wavelength of the line didn't correspond to lines
in the spectra of any known elements on Earth. Some scientists thought
it might be a new element called Coronium. Others were convinced
that it was simply the spectral signature of an already known element,
but that the wavelength of the "green line" hadn't been properly
measured. For the next 70 years solar astronomers followed eclipses
and made increasingly precise observations of the corona's spectrum.
One by one, elements like argon, oxygen, and calcium were rejected
as candidates. Finally, in 1939, the mystery was solved when Grotrian
and Edlen showed that the green line was emitted by a species of
highly ionised iron, Fe XIV.
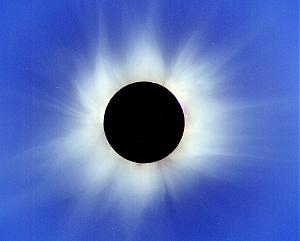
Jonathan Kern
Only in the
fleeting darkness of a total solar eclipse is the light of
the solar corona easily visible from Earth. Caltech scientist
Jonathan Kern captured this image from Romania during the
August 11, 1999, total solar eclipse. The dark sky during
an eclipse contains some blue light scattered from the bright
corona and from the sunlit horizon. The blue sky colour shows
up in this unusual picture because of the long exposure on
colour film. The coronal image was obtained using a radially
graded filter in the image plane so that the inner corona
is not over-exposed.
|
The solution of one mystery - the origin
of the green line - led to another more perplexing conundrum. Fe
XIV ("iron fourteen") is an iron atom that has lost 13 of its 26
orbital electrons. Stripping iron of so many electrons requires
an enormous amount of energy - such an ion can only exist in a very
hot gas. Careful studies of spectra obtained during eclipses show
that the temperature of the corona is about two million degrees
C, hundreds of times hotter than the surface of the Sun. Since the
corona cools rapidly, losing its heat as radiation and the solar
wind, something has to be pumping energy up from the surface. But
what? That's what scientists would like to know.
Lots of theories...Not enough data...
There are many ideas to explain the
extraordinary warmth of the Sun's corona. The leading theories fall
into three categories: miniature solar flares, atmospheric waves,
and electrical dissipation.
A typical solar flare releases 1027
ergs per second, equivalent to millions of 100-megaton hydrogen
bombs exploding at the same time. Although solar flares are powerful,
there aren't enough of them to maintain the corona's high temperature.
Instead, some solar physicists, including Ron Moore and Jason Porter
of the NASA/Marshall Space Flight Centre, suspect that miniature
solar flares, called microflares, are responsible. Microflares are
small explosions that release less than 1% of the energy in a full-fledged
flare. Although they're relatively small, microflares may be so
numerous that as an ensemble they could provide the energy needed
to keep the corona superheated.
Unfortunately, microflares are so small
that they're near the limits of what current telescopes can see.
The number of observed microflares doesn't quite add up to the energy
budget of the corona but there could be many more very faint ones
that can't yet be detected. The new TRACE satellite is providing
better sensitivity and resolution than previous instruments, and
solar physicists are looking forward to 2004, when Japan will launch
Solar B with a more powerful array of telescopes to test these theories.
Another way to heat the corona involves
magnetic waves called Alfvén waves. The solar atmosphere is permeated
with magnetic fields that are especially intense around sunspot
groups. The sun is a rumbling, boiling, dynamic place, so the magnetic
field lines are constantly shaking back and forth. These oscillations
send waves of magnetised plasma (ionised gas) propagating outward
into the corona.
"You can think of an Alfvén wave as
acting something like a bullwhip," explains Hathaway. "A magnetic
Alfvén wave starts out in the dense atmosphere near the photosphere
and moves upward into the corona where its amplitude grows in the
tenuous gas. Eventually the wave breaks and it dumps its energy
in the form of heat. It's like cracking a whip. A small shake near
your hand (the photosphere) becomes a big crack near the tip (the
corona)."
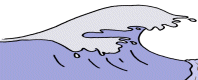
A breaking
ocean wave deposits some of its energy on the beach. Scientists
think that acoustic waves and magnetic Alfvén waves from the
sun's lower atmosphere might propagate upward into the corona
where they "break" and convert wave energy into thermal energy
that heats the gas there.
|
Solar sound waves might work in much
the same way. The Sun is churning with seismic activity that produces
a roar of gaseous compression waves in the solar atmosphere. Sound
waves that propagate from the dense lower atmosphere into the rarefied
corona break, like an ocean wave on the beach, and convert wave
energy into thermal energy.
Hathaway and colleagues have studied acoustic vibrations in the
sun's atmosphere with periods slower than about 1 minute. They find
that the total energy in such waves cannot deliver enough heat to
the corona to keep it warm. That doesn't mean that sound waves can't
do the job. The instrument that Hathaway et al used for their
study was not sensitive to vibration periods faster than about 60
seconds. If there is a great deal of energy in shorter period sound
waves and if they can propagate all the way out to the corona -
two very big ifs - then acoustic waves could be a important heating
source.
"In principle, in situ measurements by spacecraft are great
for characterising all kinds of waves, but none of the satellites
in orbit now are close enough to reveal what's really happening
in the corona," continued Hathaway. "The Solar Probe Mission could
change all that. Solar Probe will have a special heat shield that
lets it fly right through the corona within a few solar radii of
the sun. With direct measurements we might finally understand what's
going on in there."
Meanwhile, here on Earth, impatient scientists aren't waiting for
the next wave of high-tech spacecraft to solve the problem. Hundreds
of astronomers and solar physicists positioned themselves along
the path of totality of the August 11, 1999, solar eclipse to gain
a fleeting view of the corona with state-of-the-art observing hardware.
Prof. Jay Pasachoff of William's College led an expedition of over
30 scientists and students to Romania where the eclipse lasted longest,
2 minutes and 29 seconds. Using high-time-resolution digital cameras,
Pasachoff's team observed the Sun through filters sensitive to the
Fe XIV green line in hopes of detecting the signatures of high-frequency
Alfvén waves in the corona.
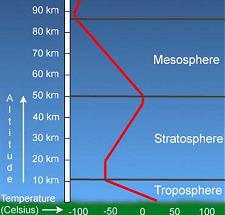
The sun isn't the only place
where temperature seems to defy common sense. Just as the
temperature of the corona increases with altitude so does
the temperature of Earth's stratosphere.
The lowest region of our atmosphere
is called the troposphere. It contains about half the total
mass of Earth's atmosphere and is the layer where weather
takes place. In the troposphere the air becomes colder as
one ascends to higher altitudes. The primary source of heat
for the troposphere is the sun-warmed surface of the Earth,
which is why the troposphere is warmest near the surface.
Just above the troposphere is the stratosphere, where the
temperature begins to increase again. The relatively high
temperature of the stratosphere is caused by the presence
of an ozone layer near an altitude of 25 kilometres. The ozone
molecules absorb high-energy ultraviolet rays from the sun,
which warm the atmosphere at that level.
|
"It was a complete and total success,"
reported Pasachoff when the eclipse was over. "We viewed the two and
a half minutes of totality in a completely clear sky. We played back
data from our hard drives, and we can see that we have fabulous scientific
data. It should keep my students and me busy for years."
Pasachoff's group was not alone. Scientists from many countries captured
high resolution images of the corona during totality in search of
microflares, magnetic vibrations and other phenomena. High-caliber
data obtained during the eclipse may help scientists evaluate another
possible mechanism for coronal heating: electrical dissipation.
It's been known since the days of Faraday and Maxwell that if you
wave a magnet back and forth in the vicinity of a conducting wire,
a current is induced in the wire. The same thing takes place in the
sun's atmosphere. Oscillating magnetic fields generate currents that
flow through the highly ionised gases above the photosphere and in
the corona. How does that heat the corona? When current flows through
a resistor some of the energy is dissipated as heat. A common light
bulb is a good analogy. Electricity moves through a partially conducting
filament, the filament glows and it also become very hot. Again, the
question hinges on better observations of magnetic fields and plasmas
in the corona. Scientists know that there is some resistive dissipation
of energy in the corona, but they can't be sure how much.
There is no shortage of ideas about what may heat the corona. Microflares,
magnetic and acoustic waves, and electrical dissipation are all good
candidates, but the observed energy flux into the corona from
each of these mechanisms is about an order of magnitude too low to
account for coronal heating. More and better data are needed to finally
reveal the culprit.
"My bet is that it's going to be some mixture," says Hathaway, "but
only time will tell! When we do know, we'll have solved one of the
big mysteries in astrophysics."
|